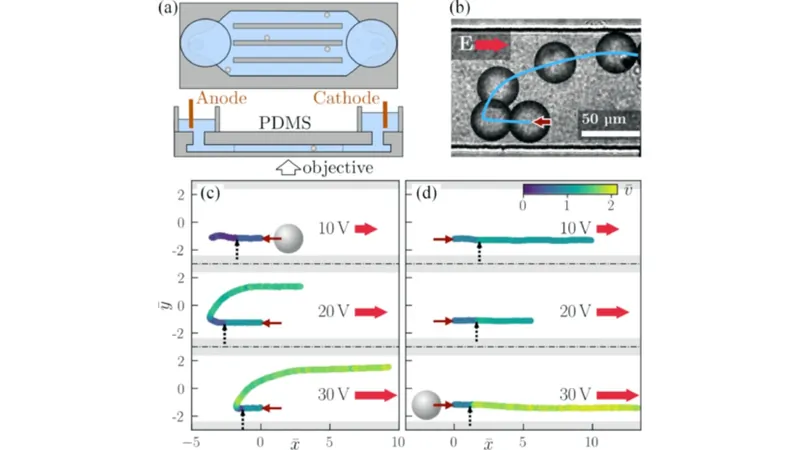
Revolutionary Breakthrough: Scientists Master the Control of Artificial Microswimmers Using Electric Fields!
2024-10-29
Author: Wei Ling
Revolutionary Breakthrough: Scientists Master the Control of Artificial Microswimmers Using Electric Fields!
In an unprecedented advancement showcased in the journal *Physical Review Letters*, researchers have unveiled an innovative technique to precisely control artificial microswimmers through the manipulation of electric fields and fluid dynamics. This breakthrough holds extraordinary promise for future applications in targeted drug delivery systems and the exciting realm of microrobotics.
Drawing inspiration from nature, such as the agility of algae and bacteria, which adeptly alter their movement in response to external stimuli like light or electric fields—a phenomenon referred to as electrotaxis—the researchers endeavored to create artificial swimmers capable of similar responsiveness.
The study's co-authors, Ranabir Dey, Assistant Professor at the Indian Institute of Technology Hyderabad, and Corinna Maaß, Associate Professor at the University of Twente, both of whom previously collaborated at the Max Planck Institute for Dynamics and Self-Organization in Göttingen, discussed the motivation behind their pioneering research. Prof. Dey expressed, “The intricate physics of active motion delivers a landscape full of fascinating phenomena distinct from passive systems.” In contrast, Prof. Maaß emphasized, “Utilizing a simplified model organisms allows us to unravel the complexities of biological systems and ultimately control them more effectively.”
Understanding Artificial Swimmers: A Deep Dive into Technology
Artificial swimmers can be categorized into two fundamental types: active colloids (commonly referred to as Janus particles) and active droplets. Their designation as “active” originates from their capacity to move autonomously in response to external or internal stimuli.
Janus particles, bearing a resemblance to the two-faced Roman deity, feature two distinct surfaces with unique chemical properties—one side may attract water while the other repels it. However, their reliance on sophisticated materials and stimuli poses challenges for practical applications.
Conversely, active droplets are simpler in nature. Composed of oil-based droplets suspended in an aqueous medium, these droplets engage in self-propulsion driven by internal reactions, allowing for greater accessibility and ease of study. They prove particularly valuable in confined environments like microchannels, which are frequently utilized in cutting-edge lab-on-a-chip technologies and microfluidic systems.
Despite their potential, the field of electrotaxis within artificial swimmers remains largely unexplored, particularly within restricted spaces involving flowing fluids. The ability to control these swimmers opens doors to remarkable applications, such as instant directional adjustments and distance scaling.
Innovative Experiments: Tracking Active Droplets in Motion
In their research, the scientists scrutinized the behavior of active droplets subjected to external electric fields within confined microchannels. The objective was to explore how external forces could influence the droplets' motion while avoiding collisions with channel walls.
Active droplets were engineered using oil droplets infused with a compound known as CB15, mixed with surfactants. When placed in microchannels outfitted with electrodes to apply electric fields, the droplets measured approximately 21 micrometers in radius. The researchers varied the voltage up to 30 volts, allowing them to control both electric field intensity and fluid pressure.
Employing high-tech video tracking systems and particle image velocimetry, they meticulously analyzed the droplets' trajectories and velocities while developing a hydrodynamic model that accounted for the droplets' surface charge and fluid interactions.
Transformative Findings in Control Mechanisms
The research revealed a remarkable range of responses from the active droplets to the manipulated electric fields. Notably, when the electric field countered the droplets’ motion, they executed perfect U-turns while their speeds increased in correlation with the electric field's strength.
By skillfully regulating both electric fields and fluid flow, the researchers successfully directed the droplets along desired paths, a concept termed electrorheotaxis. When the electric field opposed the flow, they observed reduced oscillations and steadied central movement. Conversely, when aligned with the flow, the droplets could swim upstream with modulated movements, ultimately switching to downstream flows under increased voltage.
Emerging from the hydrodynamic model, the droplets' intrinsic electric charges significantly influenced their movement dynamics. The research also revealed how channel walls affected the droplets due to interactions with surrounding fluid dynamics, confirming the model's predictive capabilities.
Prof. Dey noted, "Our findings indicate that by adjusting flow and electric field parameters, we can access a variety of motility states, including oscillations, wall motion, and reversals."
Unlocking New Frontiers: Exciting Applications Await
This groundbreaking study exemplifies how relatively simple droplets can replicate complex biological behaviors, igniting optimism for transformative biomedical applications. The incorporation of electric fields and pressure-directed flows could revolutionize how we guide cellular behavior and develop intelligent sensors, microreactors, and advanced carrier systems in medical environments.
As Prof. Maaß pointed out, these principles of guidance can extend to any charged swimmer in confined spaces, opening avenues in medical technologies, laboratory-on-a-chip systems, and bioreactors.
With researchers continuously pushing the boundaries of science, these microswimmers may soon transform the landscape of medicine and technology as we know it! Stay tuned for more groundbreaking discoveries on the horizon.