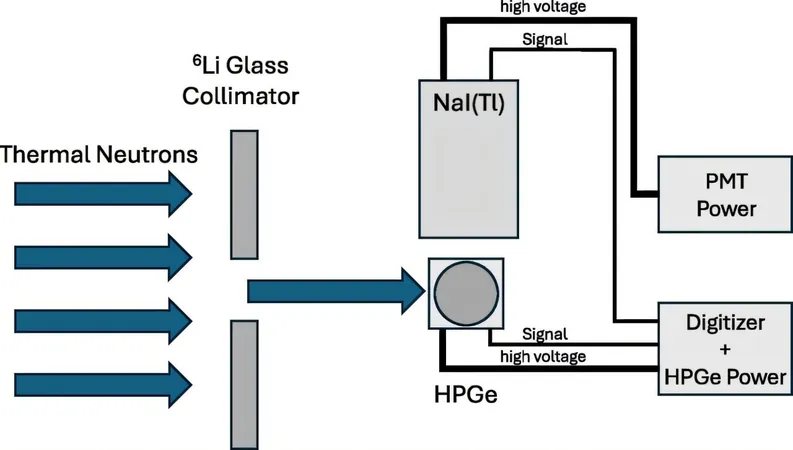
Breakthrough in Detecting Neutrinos and Dark Matter: Researchers Enhance Detector Sensitivity by 50%!
2024-11-04
Author: Sarah
Breakthrough in Detecting Neutrinos and Dark Matter: Researchers Enhance Detector Sensitivity by 50%!
In a groundbreaking advancement, researchers from the University of Michigan have significantly improved a detector's sensitivity to elusive neutrinos and potentially detectable dark matter—two key components crucial for unraveling the mysteries of particle physics and cosmology. This transformative study was published in the esteemed journal Physical Review D, marking a notable milestone in the field.
Understanding Neutrinos and Dark Matter
Detecting neutrinos and dark matter has long posed a challenge due to their extremely weak interactions with other forms of matter. Neutrinos, often referred to as "ghost particles," are minuscule subatomic entities produced during nuclear reactions in stars and as a result of radioactive decay. They possess a negligible mass—about ten million times less than an electron—allowing them to seamlessly pass through ordinary matter with little to no interaction.
The Mechanism of Detection
Detectorship of neutrinos relies on a phenomenon known as nuclear recoil. When a vast number of these particles collide with atoms, there exists a rare possibility of interaction, causing the nucleus to recoil and expelling electrons from neighboring atoms' orbits. By applying a voltage across the detector and measuring ionization energy, researchers can identify these rare neutrino collisions.
The Mystery of Dark Matter
Likewise, dark matter remains enigmatic; although it exerts a gravitational force on visible matter, it neither absorbs, reflects, nor emits light, making it undetectable through conventional means. Scientists are hot on the trail of dark matter, postulating that it acts as the “glue” binding galaxies together and shaping the universe's evolution. While elusive, the existence of hypothetical particles known as Weakly Interacting Massive Particles (WIMPs) suggests that these particles could also cause nuclear recoils similar to those potentially caused by neutrinos.
Advancements in Detection Technology
Historically, only massive underground facilities could detect these weak signals, but the landscape is changing. Researchers have utilized high purity germanium detectors (HPGe), which are compact yet powerful, measuring just a few inches in size and capable of detecting weak nuclear recoils. By leveraging the large nucleus of germanium and cooling the detector to a frigid 77 Kelvin (-196°C), they minimize atomic vibrations that could create signal noise, thus enhancing detection.
Experimental Methodology and Findings
To ensure precise measurements in their latest experiments, the researchers began by studying nuclear recoils under a neutron beam, allowing for accurate calibration. Igor Jovanovic, a professor of nuclear engineering and radiological sciences at U-M, emphasized, "Radiation is pivotal for our observations of the universe, whether it’s in high-energy experiments at the Large Hadron Collider or studies focused on dark matter. Grasping how this radiation interacts with matter greatly refines our scientific interpretations."
The research team conducted experiments to analyze the response of germanium nuclei to 254 electron volt (eV) nuclear recoils, exploring lower energy neutron beams whose interactions are not well understood. Previous studies had produced contradictory findings regarding ionization yields, necessitating a clearer understanding.
To reconcile these discrepancies, the team employed a 2 cubic centimeter HPGe detector alongside a sodium iodide (NaI) scintillation detector, capturing ionizing radiation resulting from nuclear recoils. By saving raw output data from both detectors with advanced digital recording technology, the researchers refined their analysis, removing any biases caused by signal processing. This allowed them to leverage various algorithms to pinpoint the best analytical approach.
Impact of the Research
The results were impressive—yielding a 50% increase in ionization compared to previously established theories. This enhancement dramatically boosts the sensitivity of HPGe detectors in detecting dark matter and neutrinos, paving the way for more effective experimental designs. Alexander Kavner, a doctoral graduate in the Applied Physics Program and the study's lead author, remarked, "This advancement has the potential to revolutionize commercially available detector technologies, significantly impacting ongoing neutrino research initiatives."
These experiments were executed at The Ohio State University Nuclear Reactor Laboratory, highlighting the collaborative efforts across institutions working to unlock the secrets of our universe. The implications of this research extend beyond just improved detection; it opens new frontiers in our quest to understand the unseen elements that make up nearly a quarter of the cosmos!
Looking Ahead
Stay tuned as this rapidly evolving field could change the way we perceive the universe and its mysterious components!