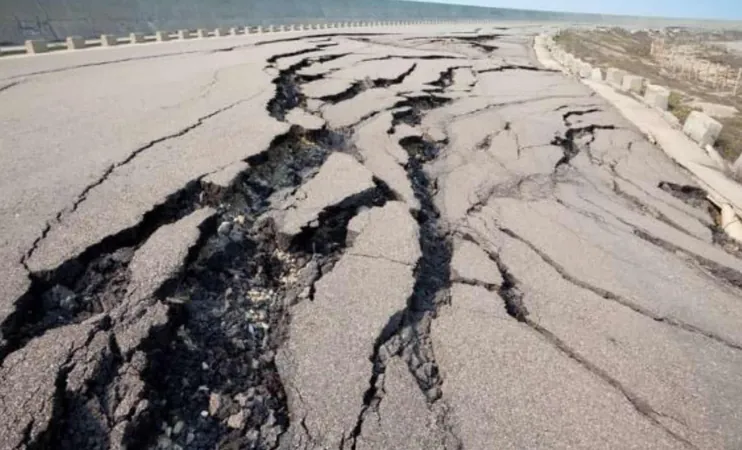
Groundbreaking Research Unlocks Secrets of Earthquake Prediction: Are We One Step Closer to Safety?
2025-01-20
Author: Chun
A revolutionary breakthrough in earthquake science has emerged from The Hebrew University of Jerusalem, promising to refine our understanding of how these devastating natural events can be predicted. Led by distinguished physicist Jay Fineberg, researchers have unveiled a previously unidentified mechanism that illustrates how stress accumulates silently along fault lines, ultimately triggering violent earthquakes. Their fascinating findings reveal the importance of slow, creeping movements—known as aseismic motion—that precede seismic activity. This discovery not only enhances our comprehension of earthquake physics but also paves the way for developing effective early warning systems that could mitigate loss of life and property damage.
Understanding the Invisible Prelude to Earthquakes
Earthquakes occur when tectonic plates become stressed at fault lines. As these plates attempt to move past one another, friction locks them into place, causing stress to build up over time. "The plates are increasingly stressed by the forces trying to move them, but are stuck at the brittle part of the interface that separates them," Fineberg explains succinctly. This critical interplay between stress and friction lays the groundwork for eventual rupture.
Fineberg's research digs deeper into the rupture process, answering the fundamental question of how an earthquake begins. "The fracture process doesn’t happen all at once. First, a crack needs to be created," he states. This initial crack forms within what is termed the nucleation zone, an area where the fault starts to weaken. As this crack grows, it transitions into a rapid rupture, releasing a significant amount of accumulated stress—a phenomenon we recognize as an earthquake.
Laboratory Discoveries: Shedding Light on Earthquake Mechanics
To unravel the complexities surrounding earthquake ignition, Fineberg and his team conducted innovative laboratory experiments. Using sheets of plexiglass to simulate fault-like conditions, they applied shear forces to mimic the movements and stresses that occur in nature. "The material composing the contacting plates will not matter," Fineberg emphasizes, alluding to the universality of the physical processes at play.
During these experiments, the researchers observed aseismic motion—stress propagation that occurs silently and without seismic wave production. This slow build-up acts as a precursor to earthquakes, with the transition from minor movements to sudden rupture being previously enigmatic. Their experiments revealed that the crack expansion behaves more like a two-dimensional patch than a linear line, as was traditionally understood. This nuance affects the energy parameters governing the rupture process.
Eventually, as the two-dimensional patch grows, the energy required for its expansion increases, causing a delay before the vital rupture occurs. The moment this patch reaches a critical state, an explosive crack motion is triggered, setting off the rapid stress release that we associate with an earthquake.
The Quest for Predictive Insights
What sets this research apart is the ability to observe the hidden dynamics of fault lines in a controlled lab environment. Fineberg remarked, "In the lab, we can watch this thing unfold and we can listen to the noises that it makes." By meticulously studying these aseismic movements and the transitions to rupture, the team hopes to identify reliable patterns or signals that could precede earthquakes.
Observing these dynamics in real-world fault lines presents a unique challenge. The research team aims to unlock the puzzle of how nature generates the cracks that lead to earthquakes—a question that remains difficult due to the prolonged periods over which these natural processes occur. The lab setting offers unparalleled opportunities to analyze these events in real time, potentially translating these findings to applications in natural fault systems.
Implications for Earthquake Prediction
The implications of this research are substantial for earthquake science and detection methodologies. By grasping the slow, invisible stress build-up and the exact conditions that trigger a rupture, scientists may soon devise tools capable of spotting early warning signs of impending earthquakes. Monitoring the aseismic motion along fault lines could offer invaluable lead time, enhancing preparedness and potentially saving lives.
Despite the promising findings, Fineberg cautions that applying this knowledge to natural fault systems requires ongoing research. Many faults undergo extensive periods of aseismic creep without resulting in earthquakes, making it challenging to distinguish between benign movements and those signaling imminent danger. Nevertheless, this breakthrough signifies a crucial advance in understanding earthquake physics.
A New Era in Seismology
Published in the prestigious journal *Nature*, the findings represent a watershed moment in the way scientists approach earthquake detection and prediction. By concentrating on the subtle prelude to seismic events, Fineberg and his team have provided critical insights that hold the potential to revolutionize our ability to anticipate earthquakes.
As research progresses, the applications of these findings may extend beyond just seismology. The principles derived from this study could also enhance the design of safer structures—ranging from residential buildings to aircraft—where understanding the dynamics of stress and rupture remains crucial.
This research not only enriches our comprehension of the mechanics behind earthquakes but also opens new doors to mitigate their catastrophic impacts. As Fineberg noted importantly, “Maybe we can uncover what you can’t really do in a real fault, because you have no detailed information on what an earthquake is doing until it explodes.”