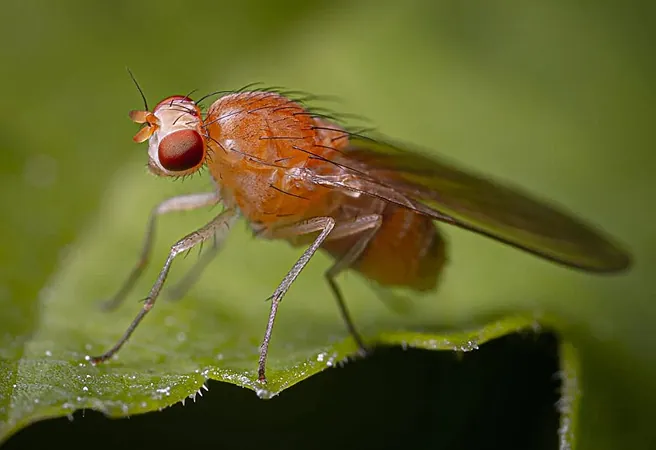
Unraveling the Secrets of Fruit Fly Development: A Revolutionary Discovery in Physics
2025-01-16
Author: Nur
Introduction
In the world of biological research, few organisms have captivated scientists quite like the common fruit fly, Drosophila melanogaster. This little creature, often seen buzzing around kitchen fruit bowls, has become a cornerstone of biological studies due to its remarkably conserved genetics and simplicity. With its short life cycle, high fertility, and genome that's been extensively mapped, the fruit fly serves as an ideal model for understanding the intricacies of life—from cellular functions to the evolutionary processes that shape us, including humans.
A Revolutionary Discovery
Recently, an exciting breakthrough has emerged from the collaboration of researchers at the University of Pennsylvania and Duke University. Led by theoretical physicist Andrea Liu, alongside Dan Kiehart and Christoph Schmidt, the team has made significant strides in understanding a specific developmental process called dorsal closure in Drosophila. This phenomenon involves the shrinking of an epithelial tissue called amnioserosa, which unexpectedly does not fluidize as anticipated. Instead, the tissue remains an elastic solid, presenting intriguing implications for condensed matter physics.
“The amnioserosa tissue is supposed to behave like a fluid as it shrinks, but our findings revealed it stays rigid, almost locked in place,” Liu explains. This discovery could hold the key to understanding broader concepts in physics, especially when looking at systems with 'tunable interactions,' a concept central to last year’s Nobel Prize-winning research.
Paranormal Physics: The Connection to the Ising Model
Liu draws parallels between the amnioserosa's behaviors and the Ising model, a foundational framework in statistical physics. The original model focused on the interactions of magnetic spins, contributing to our understanding of phase transitions—like how a material becomes magnetized. Delving into the modifications made by Nobel laureate John Hopfield, who introduced the idea of tunable interactions between spins, Liu’s research stands to bridge biological behavior and computational physics.
“Hopfield applied physical principles to neuroscience, shaping neural networks mimicking brain functions,” she continues. “By using similar concepts in our study, we can understand how the interactions between cells remain rigid during the dorsal closure process.”
Enter the Amnioserosa: A Biological Puzzle
The amnioserosa, a crucial layer during the embryonic development of fruit flies, facilitates the closing of a gap on the dorsal side as it shrinks. Despite strong expectations for the tissue to lose its rigidity, researchers found through machine learning strategies that cells largely retained their neighbor connections. “It became evident that the behavior of these cells didn’t align with traditional models,” explains co-lead author Daniel Haertter.
Manipulating conventional biophysical models, specifically the vertex model, allowed for a new understanding of how the cells preferred to shrink over time without ever turning into a fluid. The outcome of this research opens doors not only in biology but also in computational tasks, showcasing its potential to inform other fields.
A New Perspective on Condensed Matter Physics
Liu's research signifies a remarkable paradigm shift. Conventional condensed matter physics often assumes interactions between particles are static. However, Liu suggests that in biological systems—where conditions can vary and interactions are dynamic—tuning these interactions can lead to entirely new emergent properties.
“In a classic setting, adding more units doesn’t change behavior,” she says. “But here, introducing tunable interactions can drastically alter outcomes.” This realization may redefine approaches in various fields, from biology to engineering.
Through this groundbreaking study, Liu and her team's discoveries could pave the way for novel applications, translating principles of cell behavior to the design of smart materials and responsive technologies. As research expands beyond fruit flies into other biological and synthetic systems, the implications are vast and multiform.
Conclusion
In conclusion, this research not only sheds light on how complex biological movements occur in fruit flies but also propels our understanding of condensed matter physics into new territories, marrying biology and physics in ways previously unimaginable. Prepare for a future where the lessons learned from these tiny but mighty insects could lead to revolutionary advancements in both science and technology!