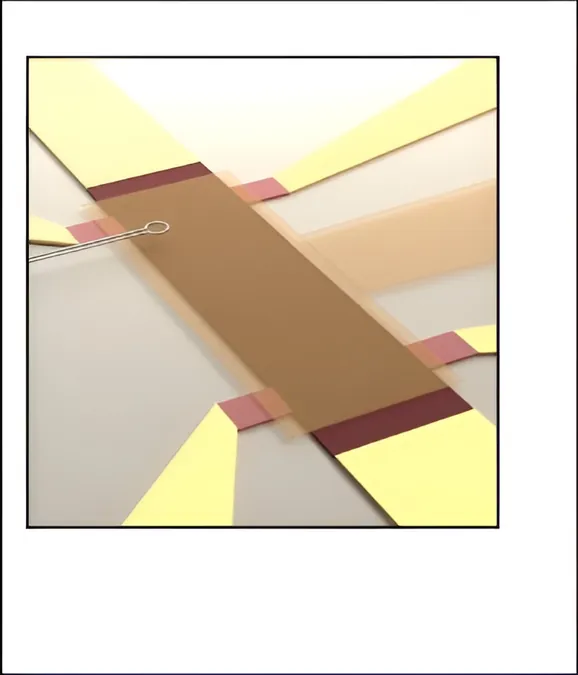
Unlocking the Secrets of Topological Censorship: A Breakthrough in Microscopic Theory
2024-09-25
The Marvel of Topological Physics
The Nobel Prize in Physics 2016 was awarded to David J. Thouless, F. Duncan M. Haldane, and J. Michael Kosterlitz for their theoretical breakthroughs involving topological phase transitions and the emergence of topological phases of matter. These topological states, unlike traditional states such as solids and liquids, can endure fluctuations and disturbances due to their unique geometric properties.
The stark contrast between conventional and topological states lies in the resilience of these exotic formations. Destroying such states would necessitate "unwinding" the intricate knots in their quantum wavefunctions — a profoundly difficult task. This robustness has been essential in the observed exactness of quantization linked to the quantum Hall effect, foundational to the field since its pioneering experiments in the 1980s, which earned Klaus von Klitzing a Nobel Prize in 1985.
The Double-Edged Sword of Topological Protection
While topological protection heralds potential for future quantum computing advancements, it also enforces topological censorship. This phenomenon restricts access to local properties of these topological states, complicating attempts to probe them with experimental analyses. Typically, experiments have only unveiled universal properties, including quantized resistance, leaving a wealth of fascinating microscopic data concealed.
Drawing parallels to a black hole, where the event horizon conceals internal properties, topological censorship ensures that even simplified models yield topologically correct results, albeit at the expense of microscopic accuracy. Common theoretical representations often suggest that current in the quantum Hall effect flows solely along the edges of material samples, a notion validated by numerous experiments.
Revolutionizing Understanding of Chern Insulators
Recent experimental endeavors from Stanford and Cornell defy this traditional view. Researchers discovered the ability to control the flow of current within what are known as Chern insulators, showcasing current that flows significantly within the material bulk, contrary to earlier assumptions. These surprising findings compelled researchers to rethink topological censorship, as now, the quantized current exhibited both edge and bulk characteristics.
The collaborative efforts of researchers from MPI-PKS in Dresden and Paris have produced a theoretical framework that successfully reconciles these observations. By defining a meandering conduction channel within the Chern insulator, their analysis aligns excellently with experimental data. Their paper posits that current need not conform to a narrow edge path but may instead occupy broader, flowing pathways akin to a winding stream.
The Experimental Landscape of Chern Insulators
Chern insulators, first predicted by Haldane in 1988, were long deemed theoretical curiosities until their experimental realization in 2009. Notably, these materials do not rely on magnetic fields to induce quantum Hall effects, adding to their versatility and appeal.
Utilizing a SQUID magnetometer, recent experiments elegantly mapped the current distribution within Chern insulators. Contrary to conventional expectations that current would be edge-bound, researchers observed electron currents permeating the material depending on external voltage parameters. This revelation presented a significant deviation from the expected outcomes, which had left a gap in theoretical understanding—an opportunity the recent theory sought to bridge.
Douçot, Kovrizhin, and Moessner's innovative approach offers the much-needed theoretical backing for the findings of the Cornell team, confirming that indeed, Chern insulators can exhibit current flow originating from within their bulk, opening up new avenues for both academic inquiry and practical applications in quantum technology.
These revelations not only enhance our comprehension of topological structures but also emphasize the importance of ongoing experimental investigations that continue to challenge traditional paradigms in the field. The journey of unraveling topological mysteries promises to be an exciting frontier in modern physics, with implications reaching well beyond the confines of theoretical research.