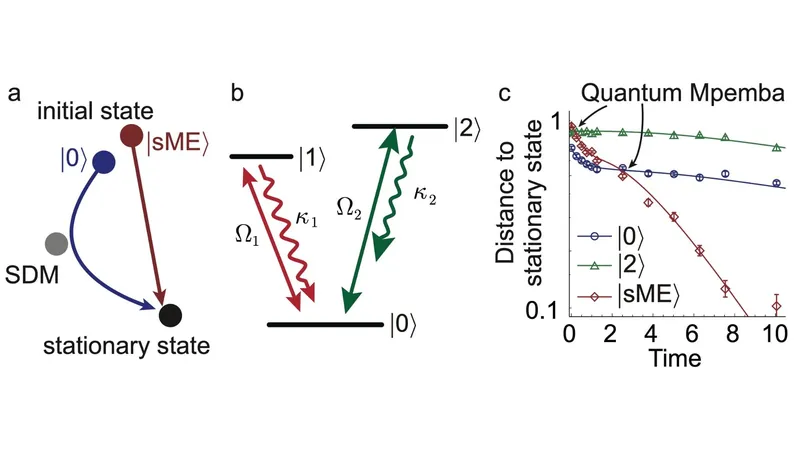
Unlocking the Secrets of Quantum Cooling: The Groundbreaking Revelation of the Mpemba Effect
2025-01-22
Author: Wei Ling
Introduction
In a captivating new study published in *Nature Communications*, researchers have taken a monumental leap into the realm of quantum physics by demonstrating the quantum version of the enigmatic strong Mpemba effect (sME) within a single trapped ion system.
The Mpemba effect, which describes the puzzling phenomenon wherein hotter water can freeze faster than colder water under certain conditions, was first noted by Tanzanian high school student Erasto Bartholomeo Mpemba in 1963. Interestingly, historical records suggest that the oddity may have been observed as far back as the time of Aristotle.
While the classical interpretation of the Mpemba effect has remained somewhat elusive to scientists, this groundbreaking research has successfully created and controlled a quantum version that operates on fundamentally different principles, causing experts to rethink our understanding of temperature dynamics at the atomic level.
Why This Matters
To gain insight into this revolutionary study, we spoke with key contributors, including Associate Professors Yan-Li Zhou and Jie Zhang, alongside Professors Hui Jing and Ping-Xing Chen from the National University of Defense Technology in China, and Associate Professor Weibin Li from the University of Nottingham. They highlighted that their collaboration centered around a common interest in quantum control.
A few years prior, the team became intrigued by research on relaxation acceleration and took inspiration from the theoretical work of Carollo et al. concerning the quantum Mpemba effect, ultimately motivating a joint experimental and theoretical exploration of this intriguing acceleration phenomenon.
Understanding the Strong Mpemba Effect
In simple terms, relaxation refers to how a system approaches its equilibrium state. In classical systems, hotter bodies are thought to decelerate faster because they have less overlap with the slowest decaying mode (SDM). However, in the quantum version, the focus shifts to relaxation dynamics at the quantum state level, evolving towards equilibrium in a distinctive manner.
The researchers demonstrated that through the preparation of an optimal quantum state—one that deliberately avoids exciting the slow decay path—an exponentially rapid relaxation can be achieved. Unlike its classical counterpart, this optimal initial state can exist as a quantum superposition, which introduces a notable distinction and is beyond the grasp of semi-classical methodologies.
The Significance of the Liouvillian Exceptional Point (LEP)
However, realizing this optimal initial state doesn't always guarantee an exponential acceleration. The research team discovered a critical juncture in their system known as the Liouvillian exceptional point (LEP), which delineates the boundary between the strong and weak Mpemba effects. At this LEP, the system's decay rates and paths coalesce into a single entity.
Before reaching the LEP, the system can indeed achieve exponential acceleration due to the avoidance of the slow decay path. However, at and beyond this point, exponential acceleration becomes unattainable, marking a significant boundary in the study of relaxation processes.
A Revolutionary Experimental Setup
To experimentally validate their findings, the researchers utilized a single trapped Calcium ion (40Ca+), focusing on three energy levels: a ground state and two excited states. By employing a highly controlled 729 nm laser and additional laser operations, they effectively prepared the ion's initial state, testing the effects of engineered relaxation dynamics.
Their groundbreaking experiment not only marked the first observation of the quantum sME but also provided experimental evidence for the coalescence of eigenvalues and eigenmodes at the LEP. “We establish a new connection between the Mpemba effect and non-Hermitian physics, paving the way for further exploration in the field,” remarked the researchers.
Beyond the Quantum Realm
The implications of understanding the Mpemba effect reach far beyond theoretical physics. The principles of energy dissipation involved in this phenomenon are crucial to many practical applications. As the researchers noted, if engineered wisely, quantum systems could exhibit enhanced relaxation properties, which could lead to faster quantum state preparations and significantly improve the performance of quantum sensors.
In summary, this groundbreaking research not only deepens our understanding of quantum phenomena but also opens the door to future advancements in quantum technology that may one day revolutionize the way we compute and sense our environment. Could this be the next big leap in quantum mechanics? Only time will tell!