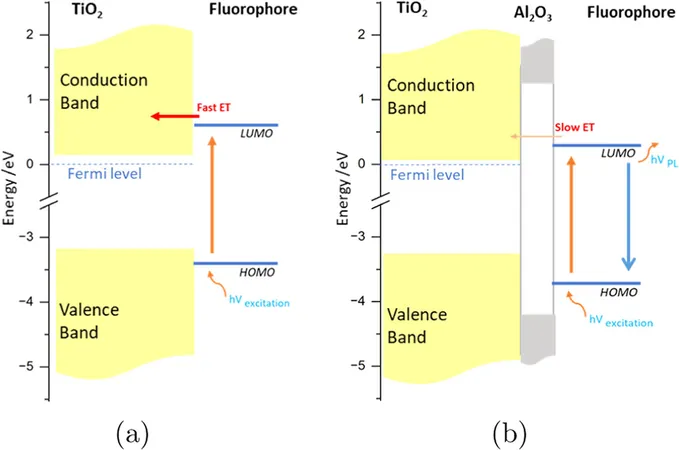
Unlocking the Secrets of Nanoscale Semiconductor Surfaces with Time-Resolved Photoluminescence
2025-03-31
Author: Yu
Unlocking the Secrets of Nanoscale Semiconductor Surfaces with Time-Resolved Photoluminescence
In the race towards advanced energy solutions and innovative sensing technologies, semiconducting metal oxides like titanium dioxide (TiO₂) have emerged as key players. Known for their abundance, resilience, and fascinating photophysical characteristics, these materials are indispensable across various applications, from photocatalysis to solar energy harvesting. However, the unpredictability of their surfaces—where most chemical interactions occur—often hinders their performance and limits their potential.
To enhance the functionality of these surfaces, researchers have developed coating strategies that involve applying ultra-thin inorganic layers. These coatings are designed to modify surface behavior without compromising the core properties of the semiconductor. But one pressing question remains: How can we observe these tiny yet significant changes at the nanoscale, especially in real-time?
A groundbreaking study from the University of Delaware, in collaboration with the Chemours Company, introduces time-resolved photoluminescence (TRPL) as a transformative optical tool to investigate the effects of coatings on semiconducting metal oxides, particularly TiO₂ nanoparticles. This innovative technique allows scientists to track the influence of inorganic surface modifiers on the photophysical response of these materials, providing crucial insights into charge transfer dynamics and surface characterization.
Understanding the Driving Forces
TiO₂ is celebrated for its wide bandgap and remarkable photoreactivity. However, its efficiency in applications is often limited by the rapid recombination of electron-hole pairs (excitons) generated upon UV light absorption—before they can be utilized in essential reactions like water splitting or pollutant degradation. This recombination is primarily a surface phenomenon, where trap states hinder charge carrier performance.
To mitigate this issue, various surface treatments have been explored, including coatings of other metal oxides such as Al₂O₃, ZrO₂, or SiO₂. These coatings aim to reduce surface recombination, enhance chemical selectivity, and modify electronic properties without altering the bulk characteristics of TiO₂.
The challenge has been in directly assessing how these surface treatments—particularly regarding their thickness and coverage—affect charge carrier dynamics at ultrafast timescales, a goal that TRPL serves to achieve.
What is Time-Resolved Photoluminescence (TRPL)?
TRPL is an advanced laser-based technique that measures the decay time of light emitted by a material post-excitation. This decay behavior provides insights into how quickly photoexcited charge carriers recombine, become trapped, or are injected into the conduction band of the semiconductor.
In this study, researchers utilized pulsed laser excitation to stimulate chromophores (light-sensitive molecules) attached to TiO₂ nanoparticles. By employing time-correlated single photon counting (TCSPC) methods, they compared the decay profiles of chromophores on bare TiO₂ versus those on surface-coated variants. This enabled direct observation of how different surface modifications impact charge transfer and recombination behavior, particularly as they relate to nanoscopic structural changes.
Key Insights from the Research
The team analyzed dye-sensitized TiO₂ samples with coatings of varying thicknesses of Al₂O₃ using a refined wet chemical deposition method that allows precise control of coating characteristics. Their TRPL measurements revealed several important distinctions among the samples:
- **Slower Decay Times**: Coated samples exhibited longer photoluminescence lifetimes compared to uncoated TiO₂, suggesting that Al₂O₃ coatings effectively passivate surface trap states.
- **Biexponential Decay**: Patchy-coated samples showed two-component decay behavior, indicating varying recombination pathways that provide a diagnostic measure of surface uniformity and quality.
- **Monoexponential Decay**: Uniformly coated samples displayed a dominant slow recombination process with decay times significantly influenced by shell thickness, demonstrating longer carrier lifetimes which are beneficial for applications in photovoltaics.
These findings underline TRPL's capability to not only confirm the presence of surface modifications but to evaluate their quality and effectiveness, marking a notable advancement in interface engineering for semiconductor applications.
Why This Matters
Grasping and controlling surface interactions in metal oxides is vital for enhancing devices that rely on charge transfer. The implications extend across multiple fields, impacting:
- **Photocatalysts**: Where surface recombination constrains quantum efficiency.
- **Dye-sensitized Solar Cells**: In which electron dynamics at oxide interfaces are critical.
- **Photoelectrochemical Sensors**: Where surface reactions dictate overall selectivity and sensitivity.
By utilizing TRPL to monitor these critical interactions, researchers can develop more effective coatings, identify suitable materials, and even observe degradation or aging effects over time.
Expanding Horizons
The implications of this research extend beyond TiO₂, encompassing a broad spectrum of wide-bandgap oxides including ZnO, SnO₂, and WO₃. TRPL provides an advantageous approach in contexts where traditional characterization methods such as XPS or TEM may falter due to limitations in time resolution, scalability, or capacity to detect minor electronic changes.
Moreover, given its nondestructive nature, TRPL can be employed in ambient or precisely controlled settings, making it ideal for in-situ and operando studies—an increasingly vital requirement in catalysis and flexible electronics.
In essence, this study elevates photoluminescence from a mere diagnostic tool to a valuable instrument for navigating charge carrier dynamics and refining functional surfaces. Through time-resolved photoluminescence, researchers can move beyond simple observations to truly understanding and optimizing semiconducting metal oxides, paving the way for the next generation of surface-driven technologies.
As advancements continue in this field, one certainty emerges: The path to clearer insights often begins with the perfect pulse of light.