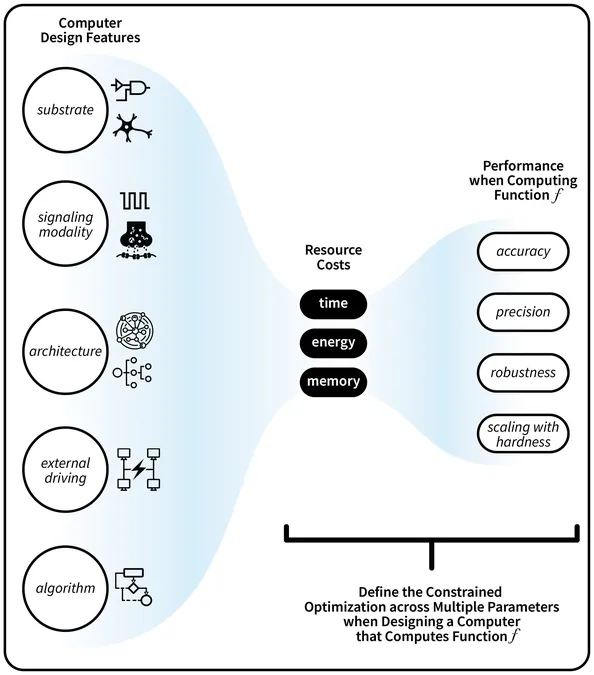
Unlocking Energy Efficiency: How Stochastic Thermodynamics is Transforming Computation
2024-10-30
Author: John Tan
Unlocking Energy Efficiency: How Stochastic Thermodynamics is Transforming Computation
In a world driven by technology, understanding the energy costs associated with computation is becoming increasingly critical. As computers process information, they operate far from thermal equilibrium, consuming energy and generating heat. A striking observation emerges: when devices like laptops completely discharge their batteries, they simply cease to function, raising an intriguing question: How does the energy required for computational tasks correlate with the specifics of those tasks?
For over a century, physicists and computer scientists have chased the elusive connection between thermodynamics and computation. Initially, it was a theoretical endeavor, but the rise of digital technology has made this relationship a pressing practical concern. Up until recently, rigorous methodologies to probe these interconnected systems were sparse.
However, a game-changing development came with the advent of stochastic thermodynamics in the early 21st century. Professor David Wolpert of the Santa Fe Institute describes it as 'a major revolution in nonequilibrium physics.' This new field provides researchers with the essential mathematical tools needed to analyze computational systems, which typically exist far from equilibrium.
In a groundbreaking Perspective published in the Proceedings of the National Academy of Sciences, Wolpert and co-author Jan Korbel outline how stochastic thermodynamics could reveal profound links between thermodynamic principles and computation. 'It gives us the means to investigate and quantify the intricate dynamics of these systems, no matter how far they are from equilibrium,' states Wolpert.
Among the critical tools offered by stochastic thermodynamics are mathematical theorems, uncertainty principles, and thermodynamic speed limits that hold true across scales, from tiny nanosystems to macroscopic constructs. Wolpert asserts that these insights were not available to the physicists of the 20th century, marking a significant leap forward in our understanding of energy dynamics.
Korbel highlights the broader implications of this research, particularly in relation to environmental concerns. 'Every computation demands energy, some of which dissipates as heat, contributing not only to warming the device but also impacting our planet,' he says. As computational energy requirements surge, mitigating these energy losses becomes paramount to achieving sustainability.
Importantly, the relevance of stochastic thermodynamics extends beyond traditional computing devices. Biological systems—from cellular processes to neural activity in the brain—similarly operate far from equilibrium and involve complex calculations. Even larger systems, such as social structures and evolutionary dynamics, are governed by the same principles.
On a practical note, gaining a deeper understanding of energy consumption in computation could lead to innovative designs for more energy-efficient technologies. Wolpert emphasizes that the findings from stochastic thermodynamics provide a unifying framework that integrates various fields of study, allowing us to connect the dots between computation, energy use, and ecological impact.
In summary, stochastic thermodynamics offers not just a theoretical view but practical tools that could reshape how we approach building the next generation of computing devices. As we grapple with the challenges of energy efficiency and the sustainability of our technological landscape, this emerging discipline stands to be a cornerstone of future innovations.