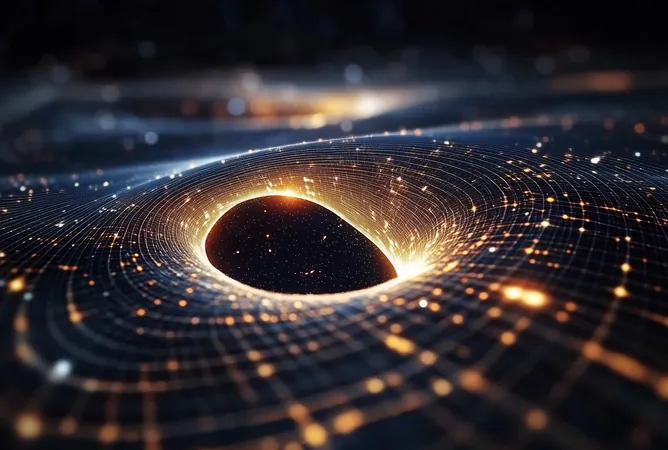
The Race to Detect Gravitons: Quantum Sensing and the Unification of Physics
2024-11-03
Author: Nur
In an exciting frontier of modern physics, researchers are ambitiously pursuing the detection of gravitons using cutting-edge quantum sensing technologies. This groundbreaking effort is not only aimed at enhancing our understanding of gravity but is also a critical step toward unifying quantum mechanics with Einstein’s theory of relativity.
Currently, advanced quantum sensors, such as those utilized at the Laser Interferometer Gravitational-Wave Observatory (LIGO), have successfully detected gravitational waves, which are ripples in the fabric of space-time caused by colossal astronomical events like black hole mergers. By employing sophisticated techniques like "squeezing," scientists are able to alleviate quantum noise—random fluctuations in measurements—which could enhance graviton detection efforts in laboratory settings.
Historically, connection between gravity and quantum mechanics has been a formidable challenge, akin to mixing oil with water. Albert Einstein's general theory of relativity, published in 1915, portrays gravity as the curvature of space-time, while quantum mechanics indicates that forces are fundamentally mediated by particles. The quest to identify gravitons—a hypothetical particle that conveys gravitational force—has long captivated physicists. If successful, this could substantiate a quantum perspective of gravity analogous to how the discovery of photons revolutionized our understanding of light.
As noted by the renowned physicist Freeman Dyson, detecting gravitons could take an extraordinarily long time—even with a detector as large as the Earth orbiting the sun. However, exciting research suggests that the integration of gravitational wave detection knowledge with quantum sensing could potentially enable us to identify gravitational signatures related to gravitons within our lifetime.
LIGO's Innovation Against Quantum Noise
At LIGO, a groundbreaking method of detecting gravitational waves is implemented. As massive cosmic events warp space-time, they induce very slight 'stretching' and 'squeezing' that can be captured by high-precision lasers bouncing between mirrors. Nonetheless, quantum noise poses a significant limitation to LIGO's sensitivity.
To counter this issue, scientists have innovated the squeezing technique, allowing them to reduce noise effectively by compromising on certain measurement precisions to enhance others. While LIGO has made strides in understanding gravitational waves, the elusive detection of an individual graviton remains a challenge yet to be realised.
Theoretical considerations reveal that cooling substantial quantum resonators to near absolute zero could allow the detection of a single graviton interacting with these resonators. This unique interaction could create measurable disturbances, providing a potential gateway to align quantum physics with gravitational theory.
Blueprint for Graviton Detection
A recent study in *Nature Communications* introduces an experimental approach that posits we are on the cusp of detecting single gravitons. The envisioned framework involves a quantum acoustic resonator made from materials such as beryllium or aluminum, significantly cooled to minimize particle interference.
Gravitational waves will be used as stimuli to induce transitions in the resonator's energy states. When a graviton interacts with this carefully crafted device, it could initiate a shift from a ground state to an excited state, yielding evidence of the graviton’s absorption.
The researchers emphasized several technological breakthroughs making this experiment viable, including advanced controls over the quantum states of sizable objects and the capacity for real-time monitoring of energy transitions. Designing high-precision resonators is fundamental in detecting energy shifts from isolated graviton interactions.
However, quantum noise remains one of the key obstacles. Researchers plan to leverage continuous sensing techniques allowing for non-invasive monitoring of energy levels to differentiate signals caused by single gravitons from other disturbances.
Challenges Ahead: Practical and Philosophical
Despite the promising framework, practical hurdles linger. Cooling resonators to their ground state is a daunting task, where thermal noise could resemble the signals we intend to capture. The existing LIGO infrastructure could complement these new detectors, correlating detected classical gravitational waves with potential graviton signals. Yet, sustaining continuous monitoring without disturbing quantum interactions presents formidable technical challenges.
Moreover, a philosophical question looms: the detection of a single graviton would not conclusively validate gravity's quantized nature. While it may indicate evidence consistent with gravity's quantum behavior, it falls short of confirming the exact quantum state of gravity itself—a gap that necessitates further exploration.
The Future of Quantum Sensing and Gravity
Regardless of the outcome regarding graviton detection, this research exemplifies how advancements in quantum technologies can propel our quest for fundamental answers in physics. Beyond exploring gravity, integrating quantum theories could yield better comprehension of cosmic phenomena like black holes and the Big Bang.
As physicists navigate this captivating yet intricate frontier, the bridge between the realms of quantum mechanics and relativity continues to narrow, hinting that remarkable discoveries may be just around the corner. Stay tuned as the scientific community races to unveil the mysteries of gravitational force and its quantum underpinnings!