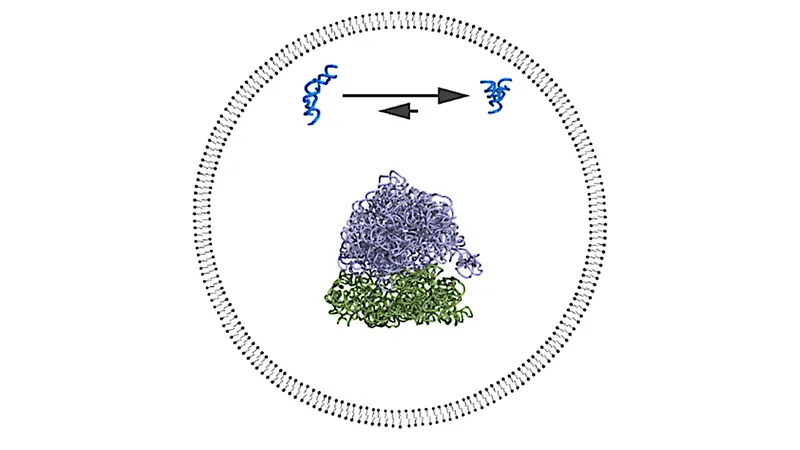
The Groundbreaking Role of Protocells in RNA Evolution and Function
2024-09-26
Creating a living system from nonliving matter stands as one of the most formidable challenges in the fields of chemistry and biophysics. Researchers draw from the early history of life, notably the concept of the prebiotic "RNA World," where all genetic and catalytic operations were presumed to be carried out by RNA itself. This original life form was considerably simpler than today’s dependent system of genetic instructions, which integrates DNA, RNA, and proteins.
To thrive and evolve, these primitive life forms likely required a mechanism for cellular compartmentalization. This has led scientists to hypothesize that minimal cells may consist of simple vesicles that enclose a prebiotic RNA metabolism. The encapsulation of RNA within these vesicles creates a unique environment, vital for studying the implications of RNA’s behavior.
The internal environment within a vesicle is marked by its closed boundaries, which significantly influence diffusion, the availability of space for macromolecules, and the effective concentration of molecules. These physical effects yield results that are distinct from chemical interactions like electrostatic repulsion that could occur at the membrane interface.
Researchers are investigating both direct and indirect interactions between the vesicle membrane and RNA, leading to fascinating "emergent" behaviors in the protocell model. Through advanced biophysical techniques, such as Förster Resonance Energy Transfer (FRET), scientists assessed how encapsulating RNA impacts its folding and functionality. They found that encapsulation usually enhances RNA folding due to the excluded volume effect, independent of chemical factors.
This stabilization effect is not merely theoretical—it translates into a significant boost in ribozyme activity, with studies showcasing improvements in various systems, such as hairpin ribozymes and self-aminoacylating RNAs. Astonishingly, researchers observed that wrapping mutant ribozymes within these protocell structures helped restore their activity, positing that encapsulation may influence not just folding and performance but also evolutionary trajectories.
To dive deeper, a high-throughput sequencing method was created, allowing the analysis of aminoacylation kinetics across thousands of ribozyme variants in unison. The findings were surprising: encapsulation tended to favor the more effective ribozyme variants, implying that during evolution, the encapsulated environment creates an uneven playing field, giving pre-existing high-activity variants an additional fitness advantage.
Rooted in Fisher’s Fundamental Theorem of Natural Selection, this increased variance in fitness is expected to expedite evolutionary adaptations, a notion confirmed experimentally. Notably, it was observed that an initially varied ribozyme population converged at a faster rate towards the most active sequences when subjected to encapsulation in vesicles.
The research elaborates on the emergent behaviors of protocells, illustrating that merely enclosing RNA in a vesicle—an occurrence that could spontaneously happen during vesicle formation—could dramatically reshape RNA’s evolutionary path.
Considering the explosive dynamics of replication and selection, even slight enhancements in RNA activity and function could lead to substantial evolutionary shifts. Through dedicated exploration of the nuanced and often complex interactions within minimal protocells, scientists aspire to unravel the pathway that bridges encapsulated RNA to the emergence of living systems.
In essence, the implications of how protocells impact RNA folding, function, and evolution hint at a thrilling frontier in our understanding of life's origins—opening doors to explore not just the history of life on Earth but also potential extraterrestrial life forms.
**Stay tuned for further updates on this exciting journey towards understanding life's beginnings!**