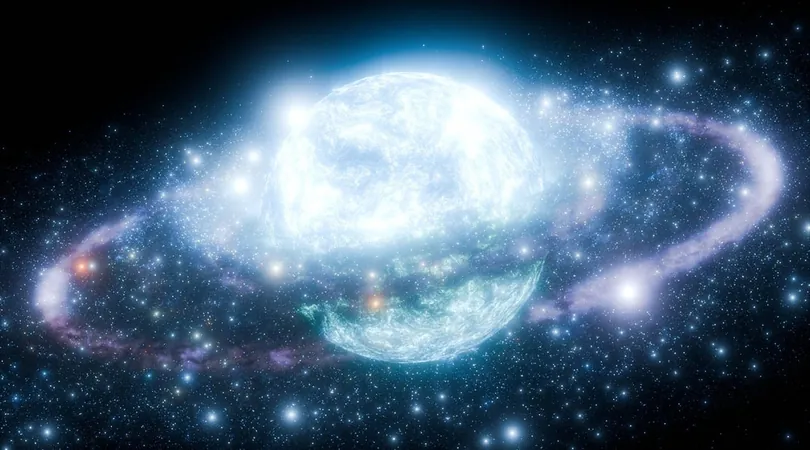
Swirling Clouds of Axions Around Neutron Stars: A Potential Breakthrough in Dark Matter Detection
2024-11-11
Author: Jia
Introduction
In a groundbreaking development, scientists have proposed a daring new method to detect dark matter by focusing on elusive radio signals emitted from neutron stars—dense remnants of monstrous stars that are predominantly composed of neutrons and possess a mass several times that of our Sun, all within a sphere measuring roughly 10 kilometers in diameter.
Dark matter, which constitutes an estimated 85% of all matter in the universe, has never been directly observed. Its existence is inferred from its gravitational influence on visible matter across vast cosmic distances. Despite numerous attempts to detect dark matter in controlled laboratory settings, these efforts have consistently fallen short.
Recent research suggests that dark matter may be clustering in dense clouds around neutron stars. This hypothesis posits that dark matter could consist of ultralight particles referred to as axions. Axions, theorized to have a mass vastly smaller than that of an electron, are strong candidates for dark matter due to their hypothesized properties that align with dark matter’s behavior in the cosmos, such as the formation of halos around galaxies.
Neutron Stars: The Axion Manufacturing Plants
Building on earlier findings, researchers revealed that the intense electromagnetic fields present in the plasma surrounding neutron stars—formed as interstellar gas is drawn in by the star's immense gravity, heated to extreme temperatures—could serve as excellent locales for axion production. The theory suggests that photons, the fundamental units of light, could readily convert into axions under these extreme conditions.
In their latest publication in *Physical Review X*, the research team delved into how axions behave once they become trapped by neutron stars. Their focus is to determine if these particles can generate detectable signals like X-rays or radio waves for our telescopes to observe.
"One innovative approach to indirectly search for axions involves monitoring for signals emanating from the neutron stars’ magnetospheres,” the scientists explained. The substantial magnetic fields and surrounding plasma enhance the interaction rates between axions and electromagnetic radiation, potentially creating a range of identifiable signatures.
A New Perspective on Axion Clouds
Although axions are a theoretical construct, their precise mass and interaction strength with electromagnetic fields remain uncertain. This study stretched beyond the known parameters to explore various possibilities.
The researchers modeled the life cycles of ten neutron stars and found that the density of axions around them could approach that of liquid water—an astonishing statistic, particularly in the backdrop of space, which averages just one atom per cubic meter.
Notably, at higher densities, axions should convert to photons more effectively, with this light potentially detected by existing observatories such as the Low-Frequency Array, the Murchison Widefield Array, and the Green Bank Telescope.
The team observed that for typical neutron stars, the density of axions near their surface could exceed the standard dark matter density by over 20 orders of magnitude during significant portions of their lifetimes. "We also highlight essential implications of axion clouds, including the emission of specific radio frequency spectral lines, indicating that current radio telescopes offer vast discovery potential,” they noted.
What Lies Ahead?
Unraveling the existence of axions through this method would be a significant milestone in astrophysics. Even if astronomers do not succeed in detecting these particles, the endeavor would help sharpen understanding of potential axion masses and their interactions with electromagnetic fields, refining theoretical models in the process.
The researchers call for further in-depth exploration of neutron star magnetospheres and the dynamics of the surrounding plasma. Moreover, better comprehension of the interactions between axions and electromagnetic fields is essential for interpreting the radio signals produced by the hypothesized axion clouds.
With these endeavors, both current and future generations of radio telescopes may soon illuminate the enigmatic characteristics of dark matter, bringing us closer to understanding one of the universe’s biggest mysteries.
Stay tuned as this fascinating research continues to unfold, offering the potential to revolutionize our grasp of the cosmos!