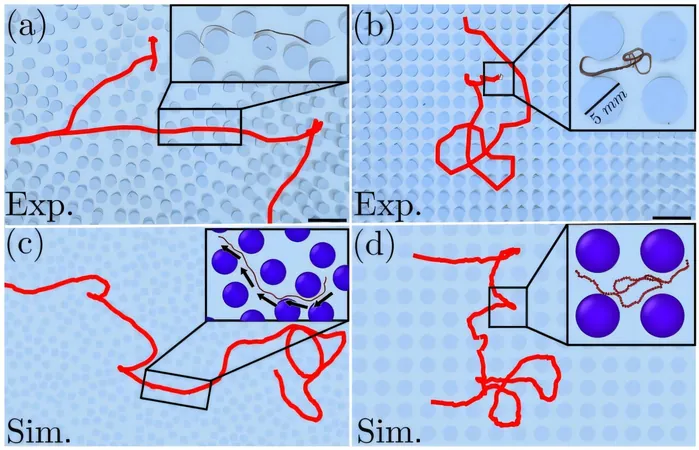
Surprising Speed: How Worms Navigate Complex Mazes Faster with More Obstacles
2025-03-27
Author: Nur
Surprising Speed: How Worms Navigate Complex Mazes Faster with More Obstacles
In a fascinating twist on what we expect from movement, researchers at the University of Amsterdam have investigated the unique locomotion patterns of worms, revealing an unexpected phenomenon: these creatures can actually move faster in crowded spaces filled with obstacles. This groundbreaking study, published in Physical Review Letters and hailed as an Editors' Suggestion, challenges our traditional understanding of movement in complex environments.
Worms, with their elongated, flexible shapes resembling spaghetti, behave like "active polymers." This means they have the ability to adapt their movement based on the surrounding environment. The research demonstrates that while one might assume more obstacles would impede movement, worms thrive in chaotic settings where they can navigate through narrow passages, effectively using their wiggly bodies to their advantage.
However, the study also uncovers complexities in this behavior. Interestingly, it was found that a higher density of obstacles doesn’t always lead to faster movement. When the obstacles are arranged in a structured manner, worms tend to slow down as the density increases, contrary to their performance in disordered environments. This outcome aligns with our expectations—an organized layout can hinder mobility as worms become slower and more entangled.
Another surprising insight involves the worms' activity level. When their activity was reduced—such as by lowering the temperature of their surroundings—they expanded their reach throughout the maze, showcasing a fascinating interplay between energy expenditure and movement efficiency. This suggests that environmental factors and internal activity levels critically influence how effectively worms can navigate through complexity.
To shed light on these observations, researchers devised a statistical model that simulates the behavior of self-driven filaments, mimicking the polymer-like traits of the worms. This model factors in the environmental structure, successfully reproducing the dynamics of worm movement. In cluttered environments, the random arrangement of obstacles creates narrow pathways that facilitate rapid movement. Conversely, in well-organized setups, the worms tend to curl up and become temporarily immobilized, leading to an overall decrease in speed.
These findings not only enrich our understanding of worm locomotion but also have broader implications. By revealing how environmental geometry impacts movement, the research can inform the development of bio-inspired robotics. Imagine robots designed to navigate through complex terrains, acting similarly to worms as they maneuver around obstacles in densely packed environments—be it in soil or even within the human body.
This study marks a significant leap in our comprehension of "active polymers," suggesting revolutionary applications that extend far beyond the natural world, paving the way for innovative designs in robotic engineering, surgery, and perhaps even new applications in medicine. As we unravel more about these remarkable creatures, we stand on the brink of breakthroughs that could redefine navigation in complex and crowded spaces. Stay tuned as we continue to explore the incredible world of biological and synthetic movement!