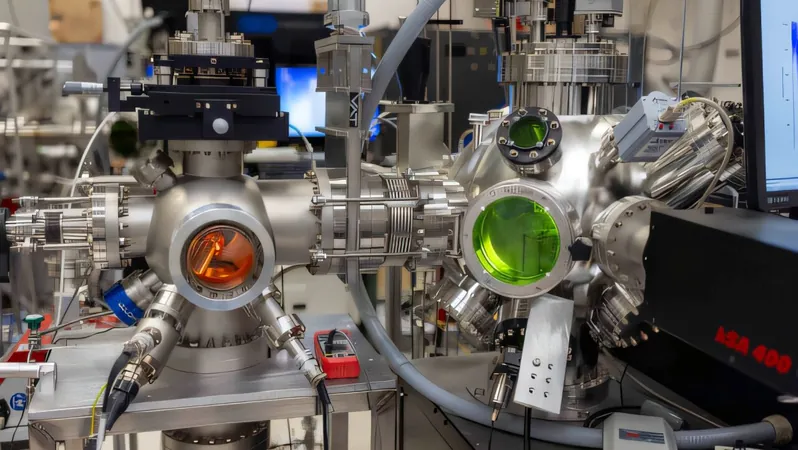
Scientists Achieve the Unthinkable: Fusion of Two 'Impossible' Materials Paves the Way for Quantum Breakthroughs!
2025-04-01
Author: Wei Ling
In a groundbreaking development that could reshape the future of quantum technology, an international research team, spearheaded by experts from Rutgers University-New Brunswick, has successfully merged two previously deemed 'impossible' materials into an extraordinary artificial structure. This remarkable synthetic quantum structure is anticipated to unlock new avenues in material science, particularly in the realm of quantum computing.
Described in an eye-catching cover feature of Nano Letters, this ambitious project unfolded over four years of tireless experimentation. Researchers developed a novel method to construct an intricate 'sandwich' structure composed of distinct atomic layers. One layer consists of dysprosium titanate—an inorganic compound not only crucial for nuclear reactors but also famous for trapping radioactive materials and hosting elusive magnetic monopole particles. The other layer is made of pyrochlore iridate, a cutting-edge magnetic semimetal that has garnered intense interest in experimental research for its unique electronic, topological, and magnetic properties.
Individually, both materials challenge conventional physics paradigms, categorized as 'impossible' due to their unique characteristics. The intersection, or interface, where these two materials converge at the atomic scale opens new scientific territories previously thought unreachable.
"This work offers a paradigm shift in our approach to designing entirely new artificial two-dimensional quantum materials," stated Jak Chakhalian, the lead researcher and the Claud Lovelace Endowed Professor of Experimental Physics at Rutgers. "The potential applications of our findings could greatly enhance quantum technologies and deepen our understanding of their fundamental properties in ways we never imagined."
Chakhalian and his team are delving into the intricate laws of quantum mechanics, a complex field that defines the behavior of matter and energy at the atomic and subatomic levels. The principles of quantum mechanics are foundational for various technologies we rely on today, including MRI machines, lasers, and even smartphones.
The contributions of several Rutgers students were paramount to the project's success: doctoral candidates Michael Terilli and Tsung-Chi Wu, along with recent graduate Dorothy Doughty, played pivotal roles in this research journey. Additionally, Mikhail Kareev, a materials scientist collaborating with Chakhalian, significantly advanced a new synthesis method while Fangdi Wen also contributed as a recent graduate in the Department of Physics and Astronomy.
The synthesis of this innovative quantum sandwich was immensely challenging, prompting the creation of a unique instrument named Q-DiP (Quantum Phenomena Discovery Platform). Completed in 2023, Q-DiP incorporates an infrared laser heater, allowing for the precise construction of materials atom by atom. This capability enables scientists to scrutinize the quantum characteristics of materials down to ultra-cold temperatures approaching absolute zero.
Dysprosium titanate, notably referred to as spin ice, supports a particularly fascinating configuration, where tiny magnetic entities known as spins mimic the structure of water ice. Within this unique configuration, magnetic monopoles—particles theorized by Nobel laureate Paul Dirac in 1931—emerge through quantum mechanical interactions, despite not existing freely in the universe.
Conversely, the pyrochlore iridate side of the sandwich houses relativistic particles called Weyl fermions. Predicted by Hermann Weyl in 1929 and identified in 2015, these particles possess extraordinary properties, moving like light and capturing various spins. Their impressive electronic stability makes pyrochlore iridate an excellent conductor, resistant to disturbances and reactive in unique ways to electromagnetic fields.
The amalgamation of these two materials creates a compelling candidate for advanced applications in quantum computing and next-generation quantum sensors. "Our findings represent a significant leap in material synthesis and could transform how we design quantum sensors and evolve spintronic devices," Chakhalian asserted.
Quantum computing taps into quantum mechanics to process information more effectively than traditional computers, utilizing quantum bits (qubits) that can exist in multiple states thanks to the principle of superposition. This groundbreaking approach allows for fast-tracked computations and sophisticated problem-solving capabilities.
The specific traits of this newly developed material hold promise for achieving complex and stable quantum states crucial for powering the next evolution in technology. When quantum technology becomes mainstream, its implications could be vast, revolutionizing fields such as drug discovery, finance, logistics, manufacturing, and even enhancing artificial intelligence systems.
As scientists continue to uncover the potentials of these 'impossible' materials, the future of quantum technologies looks strikingly bright—perhaps we are on the brink of a technological renaissance!