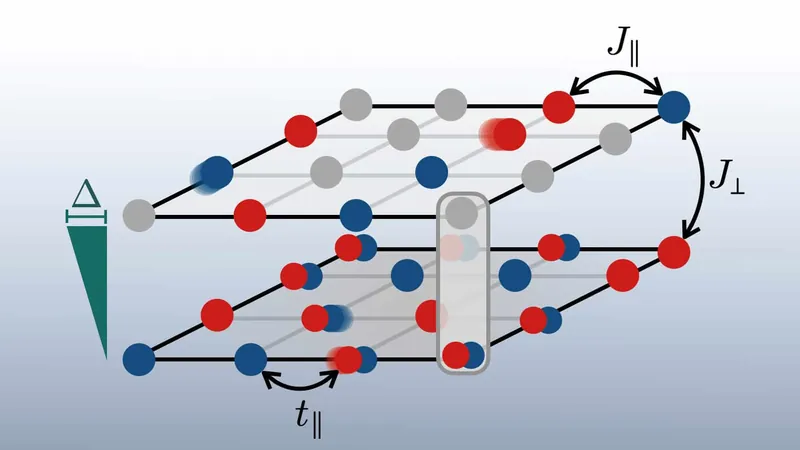
Revolutionizing Superconductivity: A Dual-Layered Laser Grid Experiment Could Hold the Key
2025-04-14
Author: Wei
Unlocking High-Temperature Superconductivity
Imagine a groundbreaking experiment that could finally reveal the mysteries behind high-temperature superconductivity! A team of innovative physicists from Germany and France, led by Henning Schlömer, is proposing a revolutionary method that harnesses a two-layered laser grid to trap atoms and delve deeper into the elusive world of superconductors.
What Makes Superconductors So Fascinating?
Superconductivity is a captivating phenomenon that allows certain materials to exhibit zero electrical resistance when cooled below a critical temperature. Despite a century of study, the mechanics behind high-temperature superconductors still baffle scientists. While we've cracked the code on 'conventional' superconductors with lower critical temperatures, understanding high-temperature varieties could unlock secrets that govern quantum phenomena in complex systems.
The Power of Optical Lattices
Optical lattices are emerging as essential tools for exploring many-body quantum systems. By overlapping two counter-propagating laser beams, researchers create a standing wave that forms a grid where atoms can be trapped. This setup allows scientists to simulate real crystalline materials, giving them the power to investigate their properties like never before.
The Challenge of Studying Electron Pairs
Superconductivity relies on long-range correlations between pairs of electrons. In laboratory settings, while the electronic properties of high-temperature superconductors can be observed, testing diverse hypotheses is tricky due to fixed properties. The flexibility of correlations within an optical lattice gives researchers an exciting opportunity to explore different models and parameters.
A Game-Changing Proposal: Dual-Layered Lattices
Schlömer and his team have crafted a novel approach by suggesting the use of a two-layered lattice system. By establishing a potential-energy difference between the layers, the atoms can only move within their respective layers, preventing any hopping between them. This configuration promotes interlayer magnetic interactions, encouraging spin correlations that can lead to superconducting behavior.
Introducing the Mixed-Dimensional Bilayer Model
Their pioneering technique is built on a ‘mixed-dimensional bilayer’ (MBD) model, which incorporates three key interactions: atomic hopping within layers, magnetic interactions between the layers, and interactions within single layers. Excitingly, numerical simulations indicate that this approach could yield superconducting behavior at significantly higher temperatures than previously understood, making these phenomena experimentally accessible.
Innovative Techniques for Measuring Correlations
To analyze pair formations within the lattice, traditional methods may fall short. Instead of adding or removing atoms, which could disrupt the lattice, the researchers propose a clever twist: doping the energetically higher layer with