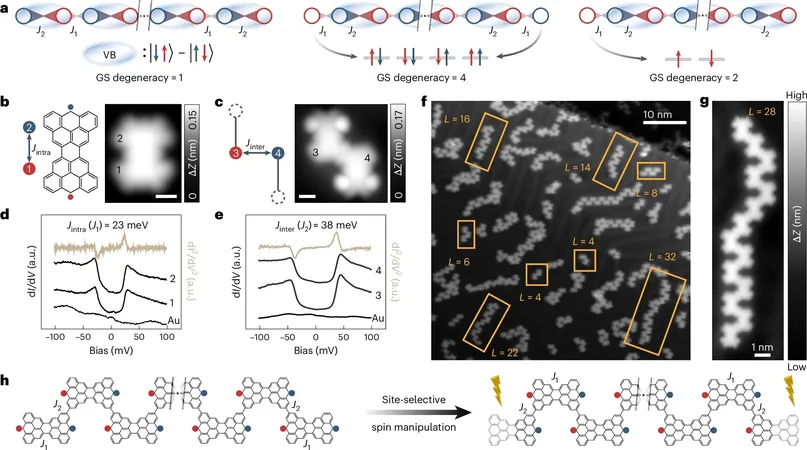
Revolutionary Quantum Model Achieved with Nanographenes: A Game Changer for Quantum Computing!
2024-10-31
Author: Sarah
In the digital realm, the basic building block of information is a bit, which can be in the state of 1 or 0. The entire scope of modern computing is based on manipulating these bits. However, in the revolutionary world of quantum computing, we encounter the qubit—capable of existing in a state of both 1 and 0 simultaneously due to a phenomenon known as superposition. This unique characteristic theoretically equips quantum computers with extraordinary computational capabilities, allowing them to tackle complex calculations exponentially faster than today's most advanced supercomputers.
Despite the theoretical promise of quantum computing, the field still grapples with many challenges, particularly in connecting qubits effectively. It's crucial because a single qubit isn't useful on its own; we need large networks of them to process information.
One intriguing avenue for creating qubit states involves the alignment of electron spins—an intrinsic property of electrons that can be likened to a tiny magnet, pointing either "up" (1) or "down" (0). The interdependence of multiple spins, known as entanglement, allows them to influence one another. However, the mathematics describing these interactions is incredibly complex, presenting significant hurdles in practical applications.
A Breakthrough in Quantum Physics
Recently, researchers at Empa's nanotech@surfaces laboratory, alongside experts from the International Iberian Nanotechnology Laboratory and the Technical University of Dresden, have developed a groundbreaking method to enable many spins to interact in a controlled manner and measure their properties with unprecedented precision. This remarkable feat has gained traction in the prestigious journal *Nature Nanotechnology*.
Their research leverages the one-dimensional alternating Heisenberg model, initially posited nearly a century ago by Nobel laureate Werner Heisenberg. Although such spin chains exist in nature, manufacturing them intentionally in materials has proven elusive—until now.
Creating Quantum Materials from Nanographenes
To construct this artificial quantum material, the researchers utilized tiny segments of the two-dimensional carbon compound known as graphene. The intricately designed shape of these nanographene molecules affects their spin properties, allowing scientists to engineer longer chains akin to quantum Lego blocks.
Specifically, the researchers employed a unique molecule dubbed Clar's Goblet, consisting of eleven carbon rings arranged in a distinct hourglass shape. Each end hosts an unpaired electron, each contributing a corresponding spin. Although originally theorized by chemist Erich Clar in 1972, Clar's Goblet was only synthesized successfully in 2019 by the same team at Empa.
By linking these goblets on a gold substrate, the researchers formed chains where molecular spins exhibit weak interactions internally while maintaining strong interactions between different molecules—a perfect manifestation of the alternating Heisenberg chain model. This capability allowed them to manipulate the chains' lengths, toggle spins on and off, and transition them between different states, delving deeper into the complex physics of their new quantum material.
From Hypothesis to Reality
The potential implications of this research are profound. Roman Fasel, the head of Empa's lab and co-author of the study, expressed his optimism, stating that this achievement could pave the way for future explorations in quantum research. He believes this synthesis technique will unlock new possibilities for constructing various quantum systems, including those with different spin configurations.
Moreover, collaboration across disciplines has been fundamental in achieving these findings. Chemists at Dresden University played a crucial role by providing the initial molecules, while theoretical insights from groups in Portugal shaped the project's direction.
As the field of quantum physics continues to evolve, the synergy between theoretical models and experimental validation will be key to turning dreamlike concepts into technological realities, positioning researchers at the cutting edge of applied quantum physics. The future of quantum computing looks brighter than ever, and this recent development perhaps hints at a time when quantum systems will achieve their full potential!