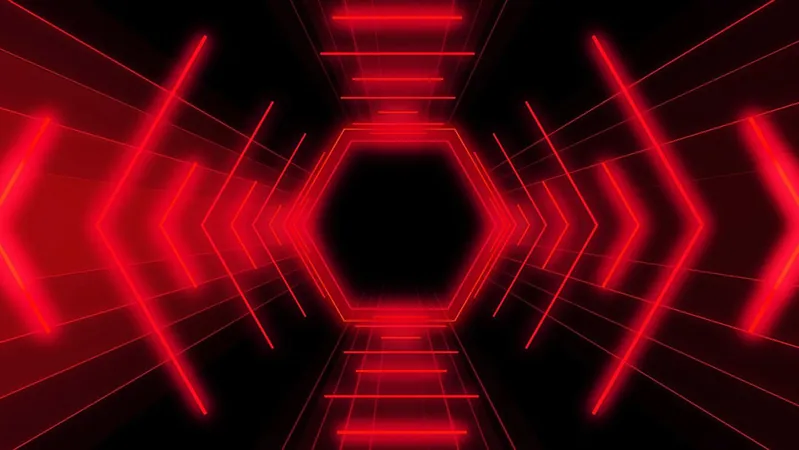
Revolutionary Electron Beams Pave the Way for Groundbreaking Advancements in Physics and Technology
2025-04-14
Author: Rajesh
A Game-Changing Breakthrough in Particle Acceleration
In a stunning advancement, researchers at the SLAC National Accelerator Laboratory have succeeded in creating ultra-dense electron beams featuring peak electric currents five times greater than ever before. By harnessing the precision of infrared laser pulses, the team is unlocking unprecedented opportunities to explore high-energy physics, replicate extreme conditions found in space, and refine new techniques in material science.
Claudio Emma, a staff scientist at SLAC and a lead author of the study, stated, "We can not only generate these powerful electron beams, but we can also customize them on demand. This opens the door to investigating a broader spectrum of physical and chemical phenomena than anything we've seen before."
From the Laboratory to the Cosmos
Electron beams are crucial in a variety of applications—from simulating nuclear explosions to sparking innovative methods for nuclear fusion. When accelerated and tightly focused, these beams heat materials to temperatures similar to those found in stellar cores or trigger rapid chemical reactions.
A standout application lies in material research, where fast electron pulses act as high-resolution cameras, capturing the instant changes in matter—like when a solid transforms into plasma or a molecule undergoes a chemical shift. However, generating such focused beams has historically been a formidable challenge.
Overcoming Technical Hurdles with Laser Precision
A key issue is that electrons in a beam do not travel at uniform speeds. This disparity causes the pulse to spread over time, diluting its intensity. Traditional beam-shaping methods, primarily using microwaves, struggle with precision, making it difficult to maintain a densely packed beam.
To tackle these challenges, the SLAC team pivoted to infrared lasers, which operate at significantly shorter wavelengths than microwaves, allowing for exceptional beam shaping. Emma emphasized, "We cannot use traditional techniques to compress electron bunches at the submicron scale without compromising beam quality. The advantage of lasers is that they facilitate a much more precise energy modulation than microwaves can offer."
A Complex Journey from Shaping to Confirmation
However, the challenge didn't stop with beam shaping. After the laser interaction in the initial part of the accelerator, the electron bunch had to travel the length of the kilometer-long facility while preserving its structure. Any fluctuations along the way could degrade the beam's performance.
Emma noted, "We have a one-kilometer-long machine, and the laser only interacts with the beam in the first 10 meters. You have to get the shaping exactly right, then transport the beam for another kilometer without losing that modulation, all while compressing it. It was no easy feat."
Confirmation: A New Era of High-Energy Physics
To validate their findings, researchers scrutinized the radiation produced by the beam and its efficiency in ionizing helium gas. The degree of ionization reflects beam density—greater ionization indicates more tightly packed electrons. Their results confirmed that the beam operated almost precisely as predicted.
A pivotal achievement was reaching peak electric currents approximately five times greater than prior techniques, a groundbreaking milestone that could revolutionize how physicists study matter and the fundamental forces of nature.
Looking to the Future: Plans for Even Greater Precision
With an eye toward the future, the team is eager to utilize lasers with even shorter wavelengths to enhance their shaping methods further. Their goal? To achieve electron pulses on the attosecond scale—allowing scientists to observe physical processes that were previously unobservable.
One of the potential explorations includes studying "filamentation"—thread-like structures found in astrophysical jets and high-energy plasmas. Emma remarked, "We know these filaments exist, but with our new capabilities, we can investigate their formation and evolution in the lab at unprecedented power levels."
An Invitation to Collaborate
The broader scientific community stands to gain immensely. The Facility for Advanced Accelerator Experimental Tests (FACET-II), where this groundbreaking study was conducted, welcomes collaborations eager to leverage these revolutionary beams.
Emma added, "FACET-II is an exciting facility for experimentation. If you’re in need of extreme beams, we have the tools for you—let’s collaborate!"
This remarkable breakthrough not only showcases the synergy of precision lasers and accelerator physics but also heralds a new era where laboratory experiments can replicate and investigate some of the universe's most extreme conditions.