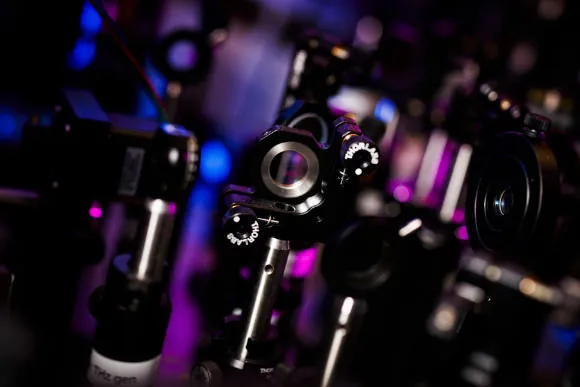
Revolutionary Discovery: MIT Physicists Use Terahertz Lasers to Control Antiferromagnetic Materials!
2024-12-26
Author: Li
In a groundbreaking study, researchers at the Massachusetts Institute of Technology (MIT) have harnessed the power of terahertz laser light to directly manipulate atoms within antiferromagnetic materials, a development that could revolutionize information processing and memory technology. This unprecedented control over antiferromagnets opens up exciting possibilities for the future of data storage.
Antiferromagnets are unique: unlike common ferromagnets, where atomic spins align in the same direction, in antiferromagnets, spins of neighboring atoms oppose each other. This “up, down, up, down” pattern cancels out their magnetic effects, rendering the material nearly unaffected by external magnetic fields—a quality that, while advantageous, has made it difficult to control these materials until now.
The implications are significant. If effective antiferromagnetic memory chips can be developed, data could be inscribed into these materials as tiny domains, where distinct patterns of atomic spins could signify binary data (0s and 1s) without being disturbed by outside magnetic influences. Such a breakthrough could mean more reliable, high-capacity data storage solutions.
A significant challenge in exploiting antiferromagnets has been finding a practical method to toggle between their magnetic states. However, MIT Professor Nuh Gedik and his team have achieved just that by using terahertz light—a kind of laser that oscillates at trillions of cycles per second. By finely tuning this terahertz light, they were able to induce a shift in the magnetic states of the antiferromagnetic material FePS3.
“We are breaking new ground here,” stated Professor Gedik. “Antiferromagnetic materials don’t respond to stray magnetic fields, which means they could be more secure and less prone to data loss.” Yet, this same insensitivity has posed challenges for researchers in developing them as controllable computing elements.
The MIT researchers suspected that by activating the terahertz light to coincide with the atomic vibrations of FePS3, they could disrupt the delicate balance of atomic spins—creating a net magnetic effect. “Picture a series of atoms like springs; when we ‘shake’ them at the right frequency, it resonates and forces them into a stable new alignment,” explained Dr. Alexander von Hoegen from MIT's physics department.
To verify their theory, the team cooled their sample of FePS3 in a vacuum chamber to temperatures below 118 K and directed terahertz pulses at it. Concurrently, they monitored the effects of two differently polarized near-infrared lasers aimed at the sample to detect changes in its magnetism. The results were stunning: the terahertz pulses consistently altered the material's state of magnetism, and this newly induced state persisted for several milliseconds after the laser was turned off—remarkably longer than similar transitions recorded in past studies.
“It’s a hot topic; light-induced phase transitions typically last for just a picosecond. We’re observing transitions lasting thousands of times longer,” noted postdoctoral researcher Dr. Batyr Ilyas. The researchers' findings were published in the prestigious journal Nature, solidifying their position at the forefront of materials science.
This stunning development not only adds to our fundamental understanding of magnetism but also paves the way for creating next-generation memory technologies that are faster, more efficient, and significantly more reliable. The implications for data storage, artificial intelligence, and computing hardware could be monumental as we step into a new era of information technology.
Stay tuned—this research could just reshape the future of how we think about data storage!