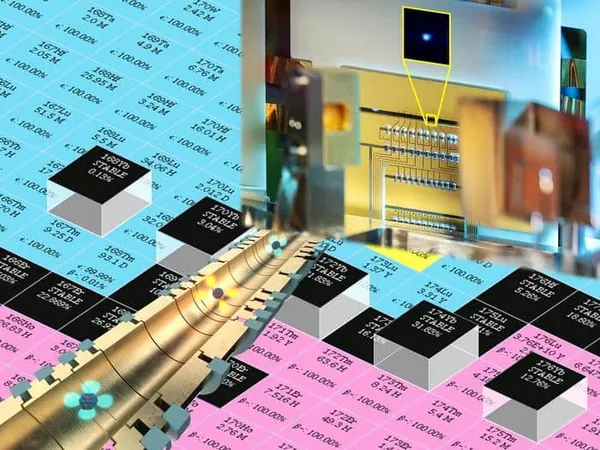
New Findings on Ytterbium Atom Anomaly: No Dark Force Required!
2025-03-27
Author: Wei
New Findings on Ytterbium Atom Anomaly: No Dark Force Required!
In a groundbreaking discovery, physicists in Germany have identified a more conventional explanation for an anomaly previously believed to indicate the existence of a mysterious “dark force.” Originally observed in ytterbium atoms, this anomaly is now understood to arise from the deformation of the nuclear structure of ytterbium isotopes as neutrons are added, rather than a new fundamental force governing the universe.
This research, spearheaded by Tanja Mehlstäubler from the Physikalisch-Technische Bundesanstalt (PTB) and Klaus Blaum from the Max Planck Institute for Nuclear Physics (MPIK), involved meticulous measurements of the energy levels and mass ratios of various ytterbium isotopes. Such measurements play a crucial role in unraveling the interactions between the particles that comprise atomic nuclei and could have significant implications for our understanding of complex phenomena such as neutron stars.
Isotopes, which are versions of an element having the same number of protons but differing numbers of neutrons, can experience shifts in their electronic energy levels based on the number of neutrons present. These shifts serve as a probing tool into the connections between electrons and neutrons, offering a window into the atomic world.
The initial anomaly was discovered in 2020 by a team from the Massachusetts Institute of Technology (MIT), which noted an unexpected deviation in the isotope shift of ytterbium. This was exciting because it raised the possibility of a fifth fundamental force acting between ordinary and dark matter, a concept that would revolutionize physics given that dark matter constitutes approximately 85% of the universe’s total matter and remains elusive in direct detection.
However, the recent research clarified that this anomaly does not hint at a new dark force. Instead, it results from the unique way the nuclear structure of ytterbium changes as more neutrons are incorporated. The team’s findings were based on high-precision measurements of the electronic state transitions of five isotopes of ytterbium—208Yb, 170Yb, 172Yb, 174Yb, and 176Yb.
To achieve this, the researchers utilized an ion trap to confine ytterbium ions while deploying an ultrastable laser to stimulate specific electronic transitions, achieving an astonishing measurement precision of 4 × 10^-9, the highest recorded so far. Additionally, they used a cryogenic Penning trap mass spectrometer to assess the atomic masses of these isotopes. By accurately determining the rotational frequencies of these ions, they could derive the mass ratios with a precision of 4 × 10^-12.
Follow-up simulations conducted by researchers at TU Darmstadt verified that the signals measured were consistent with the evolving nuclear structure of the ytterbium isotopes, rather than indicating the presence of a fifth force.
Looking ahead, the team intends to explore other isotopes of ytterbium, especially those with high or low neutron counts. This research could help mitigate uncertainties and yield even clearer constraints on potential new physical interactions. They also express interest in studying isotope chains of elements such as calcium, tin, and strontium, as continuous exploration could further test our existing understanding of nuclear matter and potentially uncover new physics.
This pivotal finding not only refines our understanding of atomic interactions but also strengthens the foundation for future explorations into the subatomic world. Could this open new doors in theoretical physics? Only time will tell!