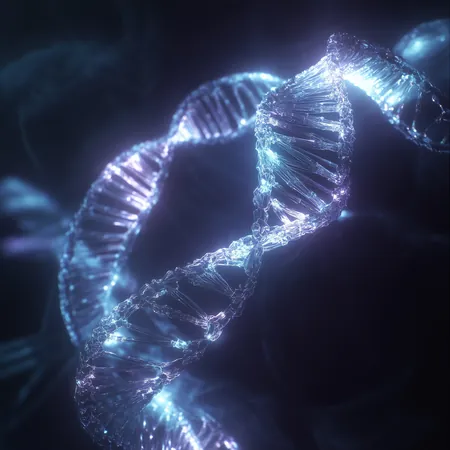
Nature's Code Decoded: Revolutionary Quantum-Inspired Genetic Algorithm Set to Transform Photonic Cooling Designs!
2024-11-14
Author: Daniel
In a groundbreaking development, researchers have unveiled a cutting-edge quantum-inspired genetic algorithm (QGA) that seamlessly integrates concepts from quantum mechanics and natural selection to revolutionize the design of photonic layer structures, particularly aimed at enhancing transparent radiative cooling systems.
The QGA significantly accelerates the exploration of complex design spaces by utilizing quantum principles such as superposition and entanglement, leading to rapid convergence on optimal configurations. This innovative approach not only promises efficiency but also minimizes computational costs by leveraging a Random Forest model that predicts material properties with greater accuracy. According to the study, this integration results in remarkable computational savings compared to traditional design methods.
The principles of natural selection, honed over millions of years, inspire this approach. Just as nature fine-tunes species traits to adapt to changing environments, the QGA employs iterative testing to refine designs, tackling challenges in material science more effectively than conventional strategies, which often involve time-consuming simulations and limited exploration.
Researchers from the University of Notre Dame, Oak Ridge National Laboratory, and Kyung Hee University published their findings in *Nature's NPJ Computational Materials*, asserting that the QGA enhances the design of multilayer structures. These photonic designs are crucial for blocking specific wavelengths of light, which can significantly reduce the need for energy-intensive active cooling systems in buildings and vehicles, especially in hot climates.
Photonic multilayer systems (PMLs) have diverse applications in optics, from photonic crystals to anti-reflective coatings, and are gaining traction for their potential in passive cooling technologies (TRC). These systems minimize heat absorption by skillfully arranging materials with properties like silicon dioxide and titanium dioxide, creating a compositional symphony that allows light to dance through while blocking unwanted heat.
Unlike traditional genetic algorithms that can fall prey to local optima, the QGA incorporates quantum mutation techniques that promote a more extensive search across the design landscape. This capability means the algorithm can evaluate multiple solutions simultaneously, adapting more effectively to the multifaceted variables at play in material design.
A significant leap forward in the study was the integration of machine learning through a Random Forest model. This advancement means that instead of laboriously running thousands of simulations, the QGA can accurately predict design efficacy with just a fraction of that computational load—just 125 simulations for the analyzed six-layer structure compared to traditional methods.
Though the results are promising, researchers caution that the QGA is still dependent on classical computational resources, which may constrain its application for highly complex problems. Future enhancements might include the integration of real quantum hardware, which is poised to elevate the capabilities and scalability of such algorithms.
This pioneering approach not only underlines the power of nature-inspired algorithms but also stands as a beacon of innovation in material science. The marriage of quantum-inspired optimization and machine learning paves the way for creating smarter, more efficient photonic structures, inviting a new era of sustainable design in technology.
As advancements continue to unfold, the QGA serves as a reminder that even the most intricate designs can benefit from the age-old wisdom of nature’s adaptations. Stay tuned, as further explorations into quantum mechanics and genetic algorithms promise to keep reshaping the landscape of photonic design technology!