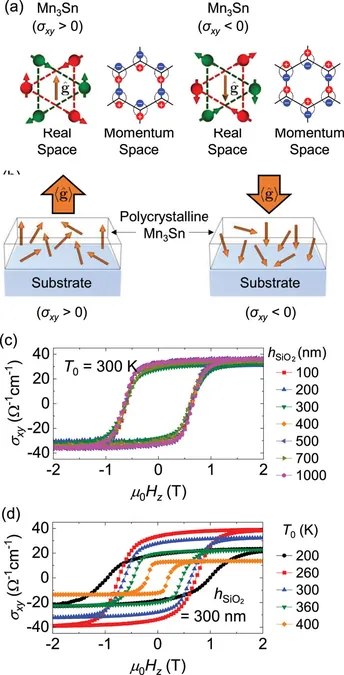
Groundbreaking Study Unveils Thermal Effects in Spintronics: A Game Changer for Efficient Computing!
2024-09-24
In an exciting leap for the future of computing technology, researchers at the University of Illinois Urbana-Champaign have made a groundbreaking discovery in the field of spintronics—devices that harness microscopic magnetism along with electric current to revolutionize data processing. This advancement, published in the journal APL Materials, is set to address a long-standing issue: how temperature influences the operation of these innovative devices.
As the demand for faster, more energy-efficient computers escalates, spintronics emerges as a promising solution, potentially offering computing speeds similar to traditional electronics, but with a significantly lower energy footprint. The pivotal question has always been whether the performance of spintronic devices diminishes under heat generated by their own operation.
The research team has developed a novel experimental technique that directly measures heating in spintronic devices, enabling an effective comparison with additional operational parameters. This breakthrough empowers scientists to identify and choose spintronic materials that maintain their magnetic properties even under elevated temperatures, paving the way for faster and more reliable devices.
Project lead Axel Hoffmann, a professor of materials science and engineering, noted, "Understanding whether the changes in magnetization arise from electrical currents or from heat is crucial for optimizing device function. Our approach brings vital clarity to the underlying physics of these mechanisms."
Unlike traditional electronics, which rely on electrical signals to store and compute information, spintronics take advantage of the electron's intrinsic spin, leading to significant energy savings. In a thrilling twist, it has been proposed that these spintronic devices, powered by rapid electronic controls, will not only maintain their energy efficiency but could also match the speed of conventional computers. Hoffmann aptly summarizes this phenomenon: "It's like getting the best of both worlds."
The challenge has been identifying suitable materials for spintronic applications. Antiferromagnets, with their unique structures characterized by alternating spin arrangements, have attracted interest due to their minimal sensitivity to adjacent devices. However, the challenge lies in controlling these spin structures using electric currents without generating excessive heat that could compromise functionality.
A pivotal debate exists within the scientific community regarding whether it is the electric current or the resultant heating that primarily influences spin alterations. Hoffmann elaborates, "Determining the driving factor impacts how we design these devices. A current-driven effect could lead to rapid operations, whereas a thermal-driven effect introduces limitations due to thermal dynamics."
Previous attempts to dissect the roles of current and temperature were stymied by the inability to accurately gauge heating in miniature devices. However, Myoung-Woo Yoo, a postdoctoral researcher in Hoffmann's team, has demonstrated a novel methodology that deduces thermal effects by observing how devices interact with substrates of varying thermal conductivities.
Yoo explained, "By placing antiferromagnetic samples on silicon dioxide substrates of different thicknesses, we can observe varying temperature responses. Thicker substrates restrict heat conduction, leading to heightened temperatures when identical currents are applied. If heating plays a crucial role in altering the spin structure, differences will emerge across devices situated on divergent substrates."
The study revealed that heating significantly affects the properties of the antiferromagnet Mn3Sn that they analyzed. There exists a spectrum of antiferromagnets still being explored for potential spintronic applications, and this innovative methodology offers a comprehensive framework to assess the interplay of heating and electrical effects systematically.
Yoo concluded, "We now have a systematic approach to evaluate the influence of thermal effects in spintronic devices. Importantly, this methodology is not only specific to spintronics but can be generalized and applied across various microscopic devices, including traditional electronics."
As this research unfolds, it ignites optimism for a new era of smart, sustainable computing, with implications that could impact everything from consumer electronics to advanced computing systems. Stay tuned—the future of technology is heating up!