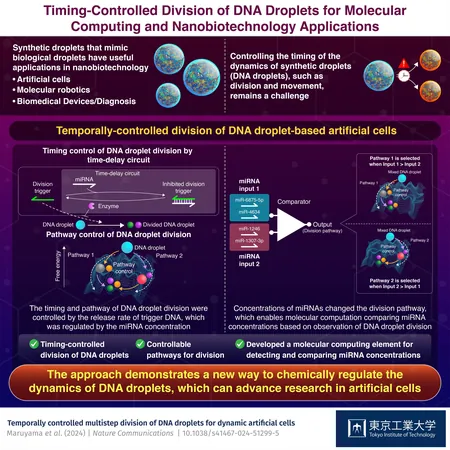
Groundbreaking Study Reveals Longevity of Synthetic DNA Droplet Division with New Timing Control Method
2024-09-26
Introduction
In an incredible leap forward for cellular biology and synthetic materials, scientists have unveiled a revolutionary method to control the timing of synthetic DNA droplet division—an advancement that could profoundly influence biochemistry, synthetic biology, and diagnostic technologies.
Understanding Biological Droplets
Biological droplets, known as liquid-liquid phase separation (LLPS) droplets, are vital components of cellular structure and function. Unlike traditional cellular structures, these droplets exist without membranes, allowing them to rapidly adapt to cellular needs. Their ability to move, divide, and alter their composition is crucial for various cellular processes, including ribosomal RNA transcription and modulating chemical reactions.
Challenges in Synthetic Applications
Inspired by the intrinsic properties of these LLPS droplets, researchers have made significant strides in creating synthetic counterparts. Despite progress in manipulating the movement and division of these droplets, precise control over the timing of these processes has presented a considerable challenge—until now.
Revolutionary Method of Control
In a pivotal study published in *Nature Communications*, scientists from the Tokyo Institute of Technology (Tokyo Tech) in Japan demonstrated a novel method for timing the division of synthetic DNA droplets. The research, led by Professor Masahiro Takinoue, employed a sophisticated time-delay circuit that regulates droplet division using a careful combination of inhibitor RNAs and the enzyme Ribonuclease H (RNase H).
Significance of the Study
Professor Takinoue emphasized the study's significance, stating, "We illustrate the timing-controlled division dynamics of DNA droplet-based artificial cells by synchronizing them with chemical reactions that showcase transient non-equilibrium relaxation processes. This allows for precise control over artificial cell division pathways."
The Innovative Approach
The innovative approach involves Y-shaped DNA nanostructures connected by six-branched DNA linkers that serve as a scaffold for the synthetic droplets. Specifically, the team engineered division trigger DNAs bound to single-stranded RNA (ssRNA) molecules known as RNA inhibitors. When RNase H degrades these inhibitors, the droplet division triggers are released, effectively stimulating the division process.
Creating Controlled Division Dynamics
“This innovative two-reaction setup generates a delay in DNA linker cleavage, which leads to precise control over the timing of droplet division,” Takinoue explained.
Pathway-Controlled Division Demonstration
The researchers successfully demonstrated pathway-controlled division within a complex droplet system containing three distinct Y-shaped DNA nanostructures. By manipulating the release of division triggers, they established two independent division pathways, showcasing the versatility and precision of their system. This breakthrough could have far-reaching implications.
Applications in Molecular Computing
Moreover, the team applied their findings to a molecular computing function called a comparator, which measures different concentrations of microRNA (miRNA). This comparator not only helped to elucidate the pathways of RNA interactions but also holds promising applications for diagnostics by facilitating a quantitative approach to compare RNA levels within biological samples.
Future Directions
While the current methods have immense potential, researchers underscore that these reactions are temporary and lack the sustained non-equilibrium states that characterize natural cellular processes. To realize long-term applications, the team emphasizes the necessity for continuous energy-supplying chemical reactions—a frontier that still awaits exploration.
Conclusion
As this field of study continues to evolve, the potential for synthetic DNA droplets is expansive, ranging from advancing our understanding of cellular functions to developing innovative diagnostic tools that could lead to breakthroughs in personalized medicine. Stay tuned as this groundbreaking research paves the way for the next generation of synthetic biology!