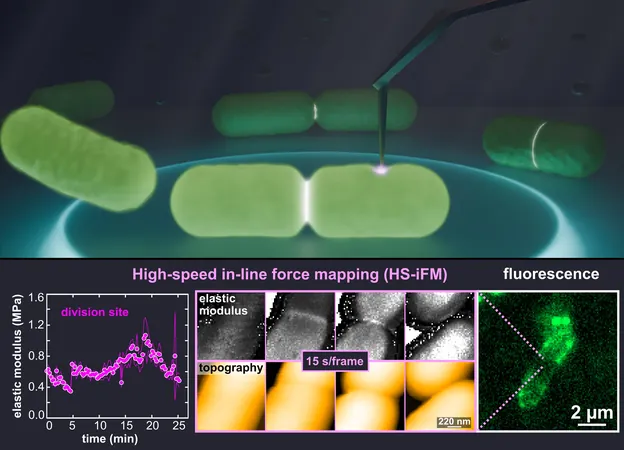
Groundbreaking Microscopy Technique Unveils E. coli's Dynamic Membrane Stiffness!
2025-01-29
Author: Arjun
Introduction to Microscopy Techniques
In the world of microscopy, both light and electron microscopy have their shortcomings. While light microscopy struggles to resolve finer details, electron microscopy can identify minute structures but requires extensive preparation, often compromising the samples' viability. Enter atomic force microscopy (AFM) — a revolutionary technique designed to probe the physical and mechanical properties of materials at high resolutions; however, its speed has hindered effective use in live biological contexts.
High-Speed In-Line Force Mapping (HS-iFM)
High-speed AFM (HS-AFM) provides faster imaging capabilities but lacks the ability to assess mechanical properties. Recognizing the limitations of existing methods, a pioneering team from the National Institutes of Natural Sciences (NINS) and Nagoya University took on the challenge to develop a new technique: high-speed in-line force mapping (HS-iFM). This innovation enables the dynamic capture of mechanical force measurements at unprecedented speeds and resolutions, suitable for living biological samples.
Research Focus on *Escherichia coli*
The research team—comprising Christian Ganser, Shigetaka Nishiguchi, Feng-Yueh Chan, and Takayuki Uchihashi—decided to focus on the common bacterium *Escherichia coli* for their initial exploration, aiming to unveil new insights into its dynamic behaviors. Their groundbreaking study has been published in the January 29 edition of *Science Advances*.
Observations During Cell Division
During their observations of *E. coli* cell division, the researchers discovered a significant stiffening of the membrane at the division site, likely attributable to localized membrane tension and cell wall thickening. “This stiffened area indicates substantial internal stresses required to deform the membrane and facilitate cellular separation,” Ganser noted.
Membrane Dynamics and Daughter Cell Formation
As the cell divided, amazing phenomena unfolded. The researchers witnessed the formation of membrane bridges between the two daughter cells, which stretched until they broke. The entire process lasted an average of 242 ± 99 seconds, offering a captivating glimpse into bacterial replication, captured in a supplementary video accompanying the research paper.
Identification of Weak Points
In a startling finding, the team identified a minuscule weak point—less than 100 nm in diameter—within a dividing *E. coli* cell. This defect caused the cell to rupture, resulting in depressurization that affected both daughter cells, implying incomplete internal separation. This discovery hints at HS-iFM's potential for precisely determining the timing of various stages in *E. coli* division and possibly that of other bacteria as well.
Potential Applications of HS-iFM
With HS-iFM, researchers can now measure both high-resolution topography and the mechanical characteristics of membranes. Observations revealed dynamic membrane holes that would appear, close, and reform while transitioning across the membrane. The team proposed that these dynamic structures might be linked to the formation of outer membrane vesicles, phenomena known to increase during cell division and the development of new cell walls between daughter bacteria.
Interesting Findings on Pore Diameter
Interestingly, the pore diameter observed—34.7 nm ± 11.8 nm—was significantly larger than the typical dimensions associated with protein complexes on bacterial surfaces, which usually measure around 8 nm. This discrepancy raises intriguing questions about the nature and functionality of these holes.
Future Directions of Research
Acknowledging the immense potential of HS-iFM, the researchers are eager to push the boundaries of this technique. “In the future, we plan to analyze how external factors, like antibiotics, influence the nanomechanical properties of living bacterial membranes,” envisioned Ganser.
Conclusion and Looking Ahead
There's more on the horizon as this team also aspires to extend HS-iFM's applications to studying transient nanomechanical properties of polymers. With aspirations to enhance both speed and resolution, they aim to visualize the mechanical behaviors of molecules down to the level of individual proteins. Stay tuned as we uncover even more revelations from this cutting-edge research that could redefine our understanding of microbial behavior!