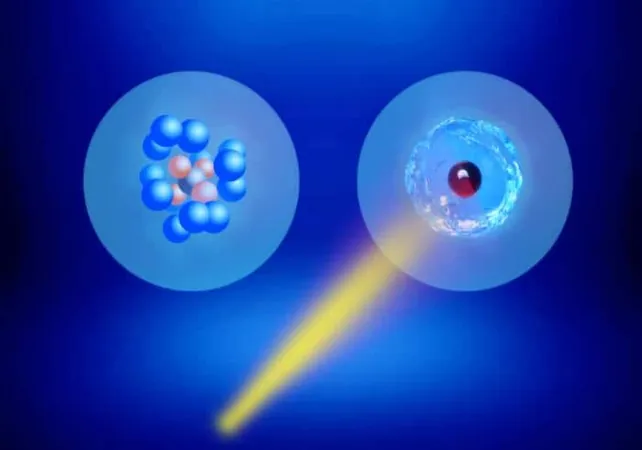
Groundbreaking Discovery: Superfluid Phase in Molecular Hydrogen Unlocks New Quantum Frontiers
2025-04-07
Author: Siti
Introduction
An international research team led by chemists from the University of British Columbia (UBC), Canada, has made a groundbreaking discovery that has the scientific community buzzing: strong experimental evidence for a superfluid phase in molecular hydrogen, successfully achieved at a chilling temperature of just 0.4 K. This remarkable achievement not only marks the first observation of superfluidity in molecular hydrogen but also sets a new benchmark in our understanding of quantum phase transitions—something akin to unlocking the secrets of the universe!
What is Superfluidity?
Superfluidity, an enigmatic quantum mechanical phenomenon, arises when certain fluids reach temperatures near absolute zero, allowing them to flow with zero viscosity and without resistance—a behavior that starkly contrasts with our everyday experiences with liquids. Until now, superfluidity had only been witnessed in helium (both 3He and 4He) and in clusters of ultracold atomic gases known as Bose-Einstein condensates. The discovery of superfluidity in the simplest and lightest molecule, molecular hydrogen (H2), could open doors to advanced applications in hydrogen storage and transport, heralding a new era in clean energy technologies.
Innovative Experimental Techniques
To navigate this challenging landscape, team leader Takamasa Momose and his colleagues employed an innovative approach. They carefully contained small clusters of hydrogen molecules inside helium nanodroplets at 0.4 K. To probe the quantum behavior of these clusters, they embedded a methane molecule as a rotating “disk” and monitored its motions using advanced laser spectroscopy techniques.
Revolutionary Findings on Superfluidity
What the team discovered was startling: in clusters with fewer than six hydrogen molecules, the rotation of methane exhibited friction. However, as the clusters expanded to ten molecules, this friction diminished significantly, letting the molecule spin faster and without resistance. This change suggests most of the surrounding hydrogen molecules operate as a singular quantum entity, indicative of superfluid behavior. Momose confirmed, “For clusters larger than N = 10, the hydrogen behaved like a perfect superfluid,” delivering a leap forward in theoretical predictions.
Future Exploration and Practical Applications
This discovery paves the way for further exploration into quantum fluids, specifically beyond helium. The team now intends to investigate larger hydrogen clusters, scaling from N = 20 to even millions, in order to dissect how superfluidity evolves in relation to size—potentially revealing whether these clusters maintain fluidity or transition into a frozen state.
Additionally, the research team aims to experiment with how superfluid hydrogen reacts under various external conditions, such as electric and magnetic fields. This could unveil even more captivating quantum phenomena, advancing our comprehension of molecular superfluidity.
On a practical level, the implications of this research are immense. Hydrogen’s role in clean energy technologies is pivotal, and gaining insights into its quantum properties could revolutionize hydrogen storage and transport methods. As Momose optimistically points out, “Understanding superfluid hydrogen may deliver critical insights for achieving frictionless flow in bulk liquid hydrogen, which is essential for more efficient energy transport systems.”
Conclusion
In summary, this unprecedented discovery not only redefines our understanding of molecular behavior at extreme conditions but also holds the promise of exciting advancements in energy efficiency and storage technologies. The future of hydrogen—both in theoretical physics and practical applications—looks brighter than ever!