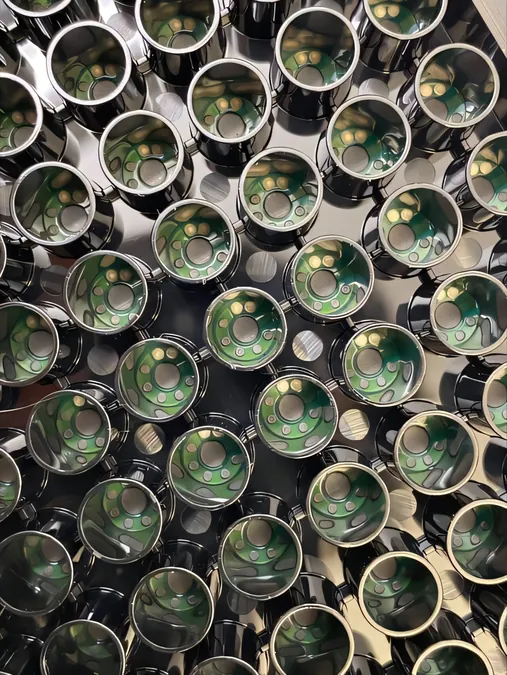
Breakthrough Technology Sheds Light on Bacterial 'Hibernation States': A Potential Game Changer in Combating Antibiotic Resistance!
2024-10-23
Author: Wei Ling
Introduction
In a groundbreaking study akin to how bears hibernate, researchers have uncovered the remarkable ability of certain bacterial cells to enter a 'low-power' metabolic state. This fascinating adaptation allows bacteria to survive without growing, which is particularly crucial in the context of antibiotic-resistant infections. A prime example of this phenomenon occurs in biofilms, which are sticky masses formed by bacteria that enable them to resist the effects of commonly used antibiotics.
Technical Challenges and Innovations
Historically, investigating bacterial behavior within these biofilms has presented significant technical challenges for scientists. However, recent advancements have led to the development of innovative lab technology that enables researchers to study the metabolic states within biofilm cores using a miniaturized liquid suspension and a highly versatile, high-throughput system.
Key Innovation: 96-Channel Potentiostat
The key innovation is a 96-channel potentiostat, a sophisticated device that measures voltage differences across tiny electrodes, allowing researchers to track the power output of each liquid well. This cutting-edge technology opens new avenues for research, particularly in understanding the intricate metabolic processes of bacteria in these challenging environments.
Research Collaboration and Findings
The research is a collaborative effort led by Dianne Newman from Caltech and Akihiro Okamoto from Japan’s National Institute for Materials Science. The results of their study, now published in the esteemed journal Cell, provide crucial insights into the bacterium Pseudomonas aeruginosa—one of the most notorious hospital-acquired pathogens responsible for various acute and chronic infections.
Focus on Phenazines and Therapeutic Applications
Newman’s laboratory has been studying Pseudomonas aeruginosa for decades, particularly focusing on small molecules known as phenazines. These molecules are essential for the bacteria’s metabolism in oxygen-deprived environments, such as the depths of biofilms. Therefore, phenazines represent a promising target for developing new therapeutic drugs to tackle stubborn infections.
The Role of the 96-Channel Potentiostat
Okamoto, known for his work in microbial electrochemistry, originally developed the 96-channel potentiostat to study another bacterium, Shewanella oneidensis. The collaboration between his lab and Newman’s has revealed exciting biomedical applications for this innovative device.
Implications for Future Research
With this powerful technology, Newman’s team can now explore pivotal questions regarding the metabolism of Pseudomonas and the role of phenazines. Extensive comparisons were made across various conditions and genetic mutants to identify the essential enzymes required for energy conservation. The results indicated that genes encoding bioenergetic pathways are critical for maintaining low-power states; the absence of these genes significantly accelerated bacterial mortality.
Conclusion
The versatility of the 96-channel potentiostat device paves the way for future studies involving diverse strains of bacteria under various conditions. 'Until now, no experimental system has allowed for direct, high-throughput measurements of metabolic power output in non-growing cells,' Newman remarks.
Final Thoughts
The implications of this research are profound, as it not only enhances our understanding of bacterial survival strategies but also holds potential for identifying novel drug targets to combat antibiotic resistance. This breakthrough could revolutionize how we tackle serious infections, making it an exciting time for the field of microbiology and therapeutic development. Stay tuned for more updates on this rapidly evolving story!