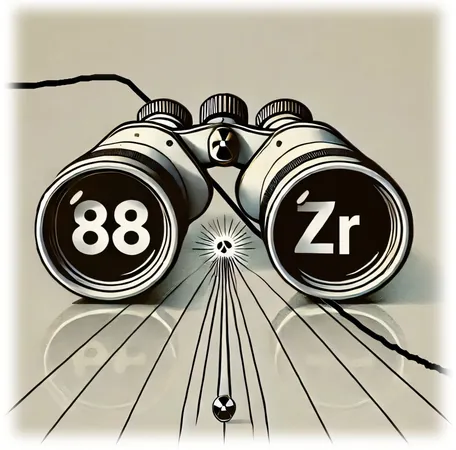
Breakthrough Study Reveals Origins of Neutron-Capture Strength in Zirconium-88, Paving the Way for Nuclear Innovations
2025-03-27
Author: Ming
Introduction
A groundbreaking study at Los Alamos National Laboratory has unveiled the origins of the remarkable neutron-capture abilities of zirconium-88 (⁸⁸Zr), a radioactive isotope with significant implications for nuclear energy and astrophysics. By employing a novel experimental methodology, researchers have resolved a longstanding mystery in nuclear physics, according to findings published in *Physical Review Letters*.
Neutron Capture Explained
Neutron capture is a pivotal process where atomic nuclei absorb neutrons—uncharged particles—and, in turn, emit gamma rays. The effectiveness of this process is quantified through the neutron-capture cross section, a measure of how likely a nucleus is to capture a neutron based on its kinetic energy. Remarkably, previous studies noted that ⁸⁸Zr possesses not only the second highest thermal cross section but also the largest resonance integral observed, a unique combination that has sparked extensive research.
Challenges in Neutron-Capture Experiments
“Neutron-capture experiments present unique challenges,” admits Thanos Stamatopoulos, the lead author of the study. “Radioactive samples emit radiation that can obscure the signals we need to detect.” Traditional methods faced limitations as gamma-ray detectors often get overwhelmed by the intrinsic radiation from the radionuclides being studied.
Innovative Methodology
To bypass these obstacles, the research team developed an innovative approach utilizing neutron transmission experiments. This methodology allows the researchers to glean information about neutron-capture processes based on how neutrons propagate through the sample, providing critical insights while avoiding interference from the radioactivity of the sample.
Pioneering Instrumentation
Moreover, the team introduced a pioneering instrument called the binocular neutron collimation system, the first of its kind designed for small samples measuring just 1 mm in diameter. This advancement holds potential for studying rare materials that are not commonly found, effectively broadening the scope of scientific inquiry in nuclear physics.
Implications of the Research
The implications of this research extend far beyond academia. The newly developed techniques and instruments could facilitate the exploration of other radionuclides, improving the prospects for nuclear power generation technologies and enhancing our understanding of astrophysical phenomena such as supernovae and neutron star mergers.
Future Prospects
Stamatopoulos further elaborated, “With the DICER instrument, we’ve opened the door to study a wide range of radionuclides that were previously beyond our reach. Our next target is the challenging ⁸⁸Y, which may lead to significant scientific advances including high-resolution measurements of 0.1 mm sample sizes and even the potential for 3D-printing radioactive samples.”
Conclusion
As this research unfolds, the possibilities for breakthroughs in nuclear science and energy advancements seem limitless, potentially shaping the future of how we harness and understand radioactive materials. Keep an eye on this space; the nuclear world is evolving fast!