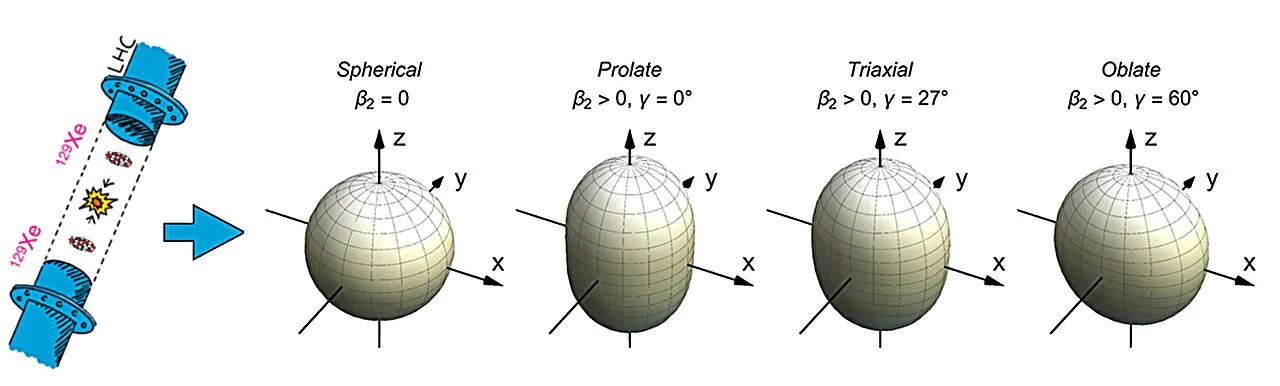
Breakthrough Research Unveils New Insights into Nuclear Shape Transitions
2024-11-11
Author: Jia
Breakthrough Research Unveils New Insights into Nuclear Shape Transitions
Groundbreaking theoretical predictions, derived from an experiment at CERN, are set to change our understanding of atomic nuclei and their transformations. A collaborative effort led by the Niels Bohr Institute of the University of Copenhagen and theoretical researchers from Peking University has shed light on previously unexplored changes in nuclear shapes during high-energy collisions.
As the study reveals, the most extreme collisions occurring in the Large Hadron Collider (LHC) are heavily influenced by the initial geometrical configurations of the colliding nuclei in their ground state. This astonishing revelation provides a pathway to uncover characteristics of these nuclei that have proven difficult to analyze through conventional methods.
The research team has outlined how variations in the shapes and fluctuations of the colliding nuclei can significantly impact the results of high-energy interactions. This marks a pivotal advancement in the scientific quest to comprehend the dynamic behavior of nuclear structures.
Leading the project, Associate Professor You Zhou from NBI emphasizes, "Our research represents a significant step forward in understanding nuclear structure, highlighting how the internal shapes of nuclei can transition."
A Closer Look at Nuclear Shapes
The study primarily focuses on Xenon nuclei, known for their distinctive oval shape akin to that of an American football. Xenon is particularly intriguing because it can oscillate between multiple configurations—spherical, prolate, or oblate—depending on the delicate balance of forces acting within the nucleus. Understanding these nuclear shape transitions not only plays a crucial role in the stability of elements but also provides key insights into the strong interactions between protons and neutrons.
The researchers conducted an elaborate eight-hour experiment at the LHC, where Xenon nuclei were accelerated to near-light speeds. These collisions generate temperatures soaring to approximately 5 trillion degrees Celsius, causing protons and neutrons to disintegrate into their fundamental components—quarks and gluons—ultimately creating a quark-gluon plasma (QGP).
Groundbreaking Findings and Future Goals
The extreme conditions generated during these experiments exist for less than 10^-24 seconds, making real-time tracking impossible. As Professor Jens Jørgen Gaardhøje explained, "We can't follow the individual steps from the collisions; instead, we analyze the resulting products and reconstruct the processes."
The team's thorough data interpretation led to the astonishing conclusion that spherical Xenon nuclei maintain a shape resembling an American football, an insight that defies the previously held belief that high-energy collisions would obliterate any identifiable characteristics.
Additionally, while the primary aim was to better understand the properties of the quark-gluon plasma created just after the Big Bang, these findings contribute significantly to nuclear shape research. "We have discovered that at ultra-high energy levels, the quark-gluon plasma behaves as a perfect liquid with negligible viscosity," noted Zhou, which opens up new questions regarding its evolution.
Advanced Methodologies and the Road Ahead
Xenon, with its 129 nucleons (54 protons and 75 neutrons), presents a complex challenge during collisions—producing approximately 30,000 smaller particles including quarks, gluons, and their antiparticles. Given the vast amount of data generated, the researchers realized that their initial method of correlating three particles was insufficient. They expanded their approach to analyze six particles, improving their ability to probe the shapes of Xenon nuclei more accurately.
To facilitate this, they developed an advanced algorithm that significantly enhances calculation efficiency, bringing sophisticated studies of quark-gluon plasma properties within reach without the need for extensive supercomputing resources.
The team is optimistic about conducting a follow-up experiment at the LHC in the summer of 2025, aiming to further explore the intricate nature of nuclear structures and the conditions of the early universe.
Stay tuned for what promises to be a captivating chapter in the ongoing saga of particle physics and nuclear research!