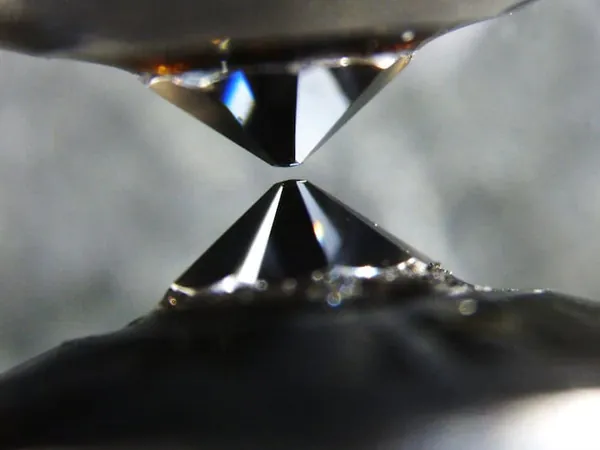
Breakthrough Discovery: First-Ever Evidence of Superconducting Gap in Hydrogen Sulphides!
2025-05-13
Author: John Tan
A Historic Milestone in Superconductivity Research
In an exciting revelation, researchers in Germany have made an unprecedented breakthrough by directly measuring a superconducting gap in a hydride sulphide material for the very first time. This groundbreaking finding serves as a "smoking gun" for superconductivity in these materials and confirms that the electron pairing fundamental to this phenomenon is indeed mediated by phonons.
What Are Superconductors?
Superconductors are remarkable materials that can conduct electricity without any resistance when cooled below a certain critical temperature (Tc). While many superconductors require chilling to extremely low temperatures—like solid mercury, which has a Tc of just 4.2 K—scientists are fervently searching for materials that can superconduct at much higher temperatures, ideally room temperature. Achieving this could revolutionize electrical systems, greatly enhancing the efficiency of generators and transmission lines.
The Evolution of High-Temperature Superconductors
The quest for higher-temperature superconductors gained momentum in the late 20th century, particularly with the discovery of high-temperature copper oxide superconductors in the 1980s and 90s, boasting Tcs between 30-133 K. However, a major leap occurred in 2015 when researchers discovered that H3S could superconduct at a staggering 203 K under extreme pressures of 150 GPa.
This discovery ignited a new wave of interest in hydrogen-rich materials. Then, in 2019, lanthanum decahydride (LaH10) shattered previous records with a Tc between 250-260 K, again requiring extremely high pressures. By 2021, cerium hydrides further evolved the field, showcasing stable high-temperature superconductivity at lower pressures (approximately 80 GPa) with innovative compounds like CeH9 and CeH10.
Understanding the Mechanism: How Do Superhydrides Work?
One lingering question about superhydrides has been their superconductivity mechanism. According to the established Bardeen–Cooper–Schrieffer (BCS) theory, superconductivity arises when electrons overcome their natural repulsion to form pairs, known as Cooper pairs. These pairs can travel freely through the material, creating a supercurrent without interruptions from phonons or impurities.
Central to identifying a material's superconducting nature is the energy gap near the Fermi level, indicating how much energy is required to break apart the Cooper pairs. However, measuring this gap in superhydrides has proven challenging due to the extreme pressures needed for their superconducting state.
Innovative Spectroscopy Under Extreme Conditions
Now, a pioneering team from Germany's Max Planck Institute for Chemistry has developed a new form of spectroscopy capable of functioning under these extreme pressures. They introduced planar electron tunneling spectroscopy, which involved creating highly pure planar tunnel junctions of H3S and its deuterated form, D3S, at pressures exceeding 100 GPa.
Using laser heating techniques, they composed junctions containing tantalum (the metal), tantalum pentoxide (the barrier), and the superconductors. Their measurements revealed superconducting gaps of 60 meV for H3S and 44 meV for D3S. As researcher Feng Du noted, the smaller gap in D3S confirms that superconductivity in H3S results from electron-phonon interactions, backing long-standing theoretical predictions.
Looking Ahead: The Future of Superconductivity
This groundbreaking research not only opens the door to understanding superhydride materials but also paves the way for future innovations in superconductivity. The implications could reshape electronics as we know them—who knows, we might soon witness the rise of room-temperature superconductors!