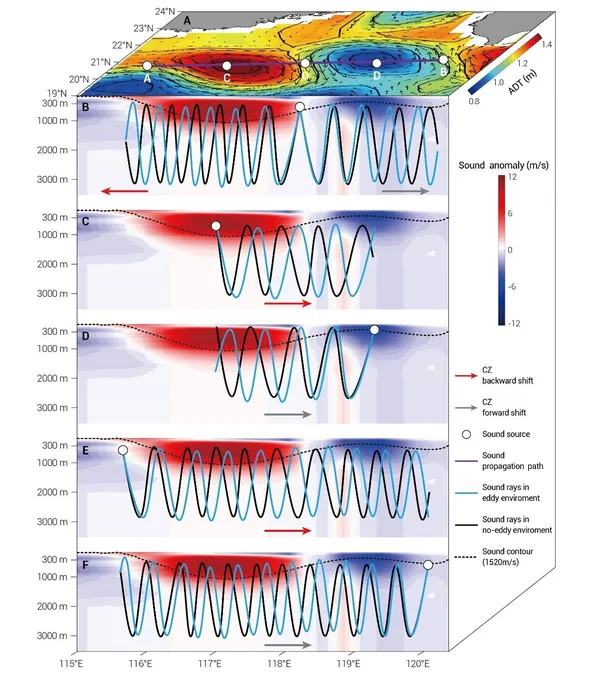
Breaking Waves: How Ocean Currents Shape Sound Travel Beneath the Surface
2025-04-16
Author: Arjun
Sound waves traverse various mediums, and when it comes to ocean waters, their journey can be profoundly influenced by the currents beneath the surface.
Mesoscale eddies, those colossal, swirling currents stretching kilometers across, are fundamental players in the ocean's dynamics. Unlike permanent currents like the Gulf Stream, these eddies have a life span of weeks to months and significantly impact climate, nutrient distribution, and even ocean circulation.
But here’s the twist: these temporary eddies also dramatically alter how sound waves propagate, dependent on their size, speed, and direction. Understanding this interplay is vital, especially for tasks like underwater navigation and long-range communication.
While the effects of single mesoscale eddies on sound have been meticulously documented, the intriguing interaction between pairs of opposing eddies remains less understood.
In the northeast South China Sea, a hotspot for anticyclonic eddies (those that spin clockwise), researchers spotted a common companion: the cyclonic eddy (which spins counter-clockwise). Together, they form exciting dipole eddies that potentially affect sound transmission.
A pivotal study led by scientists from the Chinese Academy of Sciences and Shandong University of Science and Technology shed light on the acoustic ramifications of these dipole eddies. Their findings, detailed in Ocean-Land-Atmosphere Research, uncover fascinating sound-speed configurations and propagation patterns.
"For the first time, we systematically explored the acoustic impact of pairs of dipole eddies in the northeastern South China Sea," remarked Lu Lu, lead researcher from the Institute of Oceanology.
The team's primary goal was to investigate how variations in temperature and salinity—thanks to dipole eddies—alter sound-speed profiles at varying depths, ultimately affecting sound wave behavior.
Harnessing satellite altimeter data and advanced modeling techniques, researchers were able to predict sound propagation through these dynamic eddies.
Their discoveries revealed that anticyclonic eddies often sink at their centers, leading to lower temperatures and salinity on one side, while cyclonic eddies promote water upwelling, enhancing both temperature and sound speed.
The conditions within these dipole eddies contrast sharply with surrounding waters. Based on historical data from 2000 to 2020, the team calculated significant anomalies in temperature, salinity, and sound speed.
The research also highlighted a crucial insight: warm-core anticyclonic eddies create positive sound speed anomalies, while cold-core cyclonic eddies induce negative anomalies. This distinction shifts the convergence zones (CZs), affecting sound intensity and acoustic transmission loss.
Through their modeling efforts, the team demonstrated that dipole eddies alter CZs and transmission loss, regardless of the sound source’s position, whether nestled in the dipole, centered on an eddy, or adjacent to them.
Despite these advancements, the researchers noted limitations in their study design. The dipole eddies’ southwestward movement in the South China Sea might have skewed the results, and simulations assumed a flat-bottomed terrain—which could also distort sound wave propagation.
Looking ahead, Lu shared ambitions for future studies to assess the impact of dipole eddies during their evolution and under realistic terrain conditions. The ultimate aim? To distill the overarching principles governing how multiple mesoscale vortices influence sound wave propagation in the depths of the ocean.