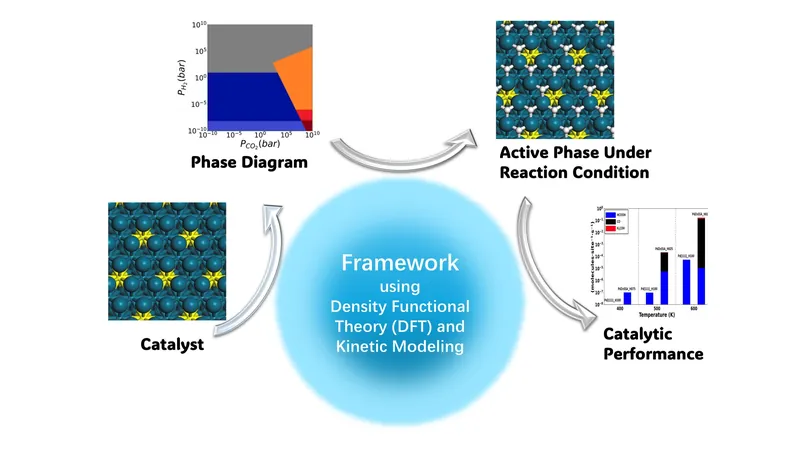
Unlocking the Secrets of Catalytic Selectivity: New Theoretical Framework Paves the Way for Better Processes!
2024-10-22
Author: Olivia
Introduction
In a groundbreaking study at Brookhaven National Laboratory, researchers have unveiled a revolutionary theoretical framework that enhances our understanding of catalysts—essential components that accelerate chemical reactions by lowering energy barriers. This innovative approach not only promises to refine catalytic performance but also to guide the design of more effective catalysts for various industrial applications.
Key Findings
The research, published in the prestigious journal *Chem Catalysis*, demonstrates how crucial reaction conditions—such as temperature and pressure—can significantly influence a catalyst's structure, operational efficiency, and the variety of products it yields. Ping Liu, a prominent theorist from Brookhaven Lab's Chemistry Division and an adjunct professor at Stony Brook University, emphasized the importance of their findings: "Our results highlight the significant impact the reaction environment can have on catalytic performance."
Focus on CO2 Conversion
The study specifically focuses on catalysts that facilitate the conversion of carbon dioxide (CO2)—a major greenhouse gas—into valuable products like methanol through hydrogenation reactions. Interestingly, previous research had shown that palladium (Pd) catalysts tended to produce formic acid (HCOOH), while theoretical predictions suggested methanol (H3COH) was a more likely product, sparking confusion within the scientific community.
Innovative Modeling Techniques
To resolve this discrepancy, Liu's graduate student, Hong Zhang, employed advanced modeling techniques based on density functional theory and kinetic modeling. This framework effectively captures the dynamic behavior and structural changes occurring in catalysts during real-time reactions. By mirroring experimental conditions through computational modeling, the team was able to pinpoint how different reaction environments affect catalytic behavior.
Key Discovery
One significant discovery was that as temperature rises, the hydrogen coverage on the catalyst surface decreases, which, in turn, facilitates more efficient reactions. "At higher temperatures, we observed a shift in selectivity, moving from formic acid production to generating greater amounts of carbon monoxide and methanol," Liu explained. The researchers identified that these active sites transformed from single hydrogen vacancies to larger clusters, enhancing methanol production—a key breakthrough in addressing the theoretical-experimental gap.
Versatility of the Framework
Furthermore, this comprehensive framework was tested on various catalysts, including pure palladium and bulk alloys with zinc and silver, demonstrating its versatility and effectiveness in predicting catalytic behavior accurately, while also reducing computational costs.
Future Implications
Liu expressed confidence that this theoretical framework not only advances our understanding of CO2 hydrogenation reactions but also establishes a foundation for exploring other types of catalytic processes. "It provides a method for delving deeper into the active sites of catalysts under operational conditions," she noted, highlighting its broad implications for enhancing catalyst design in the future.
Conclusion
With the ongoing need for sustainable energy solutions, innovations like these are crucial. The research team at Brookhaven Lab continues to bridge the gap between theoretical modeling and experimental validation, laying the groundwork for superior catalysts that can meet the demands of a greener and more efficient industrial landscape. Stay tuned to see how this exciting development will transform the future of chemical engineering and contribute to a sustainable world!