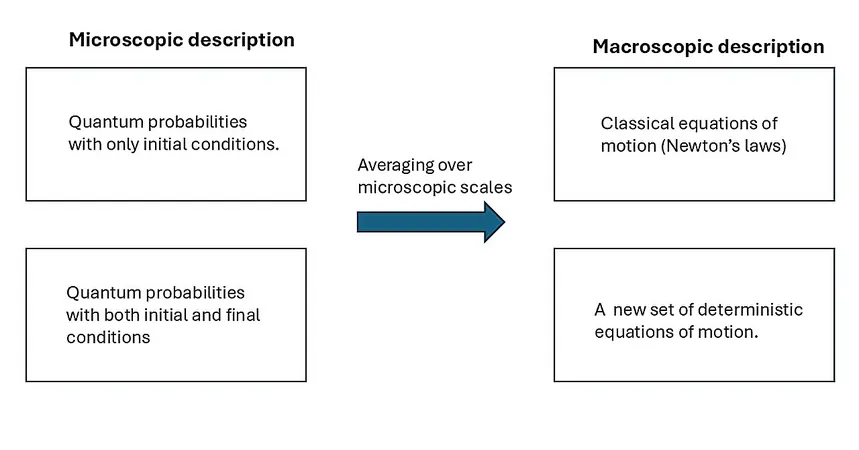
New Quantum Cosmology Insights Could Unravel the Mystery of the Universe's Accelerated Expansion!
2025-04-07
Author: Charlotte
New Quantum Cosmology Insights Could Unravel the Mystery of the Universe's Accelerated Expansion!
In the realm of cosmology, the accelerated expansion of our universe presents one of the most perplexing challenges for scientists. Recent investigations into the intriguing concept of teleology—essentially a framework suggesting that certain natural processes are directed towards an ultimate goal—might offer groundbreaking insights into this cosmic puzzle.
Traditionally, teleological viewpoints have been dismissed by many in the scientific community as outdated and incompatible with modern physics, primarily due to their untestable nature. However, a fresh perspective that encompasses quantum theory reveals considerable room for teleology by incorporating final conditions as intrinsic attributes of quantum cosmology, which treats the universe as an entire quantum system.
In this context, new research scrutinizing final conditions in quantum cosmology has illuminated a pathway toward understanding the universe's accelerated expansion without invoking new physics. While established theories frequently attribute this phenomenon to a minuscule yet non-zero cosmological constant, mysterious dark energy, or drastic modifications to gravity theories, the findings from quantum cosmology suggest an alternative explanation centered on final quantum conditions alone.
Historically, physicists have addressed time evolution as an initial value problem, aligning with Newtonian physics principles. However, the singularities present in general relativity complicate this narrative, as the Big Bang singularity creates a situation where initial conditions cannot be defined to predict future outcomes.
The exploration into quantum cosmology aims to resolve these singularities through quantum effects, albeit presenting its unique challenges given that the conventional causal evolution observed in classical physics does not apply to quantum states. In quantum theory, states often function merely as informational constructs guiding probabilistic outcomes. Yet, recent developments indicate that the concept of final quantum states can vastly enrich our understanding of cosmological processes.
Remarkably, certain interpretations of quantum probabilities have been successfully applied experimentally through a process known as post-selection. Here, only even runs of experiments meeting specific final conditions are assessed. In quantum cosmology, the universe is treated as a coherent quantum system, viewing each initial and final state as integral components of a fundamental probability assignment that governs the universe's birth and death.
While most quantum cosmology research emphasizes initial states, notable exceptions, such as Stephen Hawking’s suggestion of correlated final conditions for a potential Big Crunch, have surfaced. However, with cosmic acceleration firmly established since the late 1990s, scenarios predicting a Big Crunch have lost credibility.
The recent analysis delved deeper into deriving deterministic quantum probabilities while incorporating both initial and final conditions. This exploration yielded novel equations that mirror classical physics mathematics, yet they stand largely devoid of reliance on speculative theories of quantum gravity. The conclusions, published in Physical Review D, assert that these teleological quantum cosmological models consistently depict a universe transitioning from non-accelerating to accelerating phases, aligning with contemporary astronomical observations.
Critically, this framework eliminates the need for a cosmological constant, dark energy, or modifications to gravity. Rather, cosmic acceleration emerges fundamentally from quantum-level final conditions, presenting it as a significant macroscopic quantum effect.
As promising as these findings may be, further research is essential to benchmark teleological quantum cosmology against alternative models of cosmic acceleration. One noted limitation is that the deterministic approach struggles near the Big Bang, thereby necessitating a comprehensive quantum description to probe the early universe effectively.
Investigating simplified models may provide valuable insights into the early universe's cosmological inhomogeneities, ultimately connecting these insights with empirical data from the cosmic microwave background and primordial gravitational waves.
In conclusion, while the mysteries of the universe's expansion remain vast, the exploration of final states in quantum cosmology may serve as a pivotal step toward unlocking answers that challenge our understanding of existence itself. Is it possible that the answers to our cosmic questions lie within the quantum world, waiting just beyond our current comprehension?