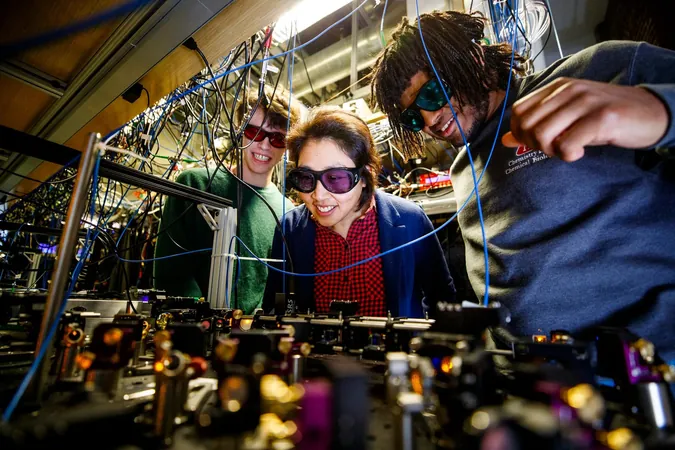
Breakthrough in Quantum Computing: Scientists Trap Molecules to Execute Complex Quantum Operations!
2025-01-21
Author: Amelia
Breakthrough in Quantum Computing: Scientists Trap Molecules to Execute Complex Quantum Operations!
In an astonishing first, a team of scientists from Harvard University has successfully trapped molecules to perform quantum operations, paving the way for unprecedented advancements in quantum computing. Historically, quantum computing has largely been dominated by simpler particles like ions and atoms, as molecules were deemed too complex and unpredictable for reliable quantum operations.
This breakthrough hinges on the utilization of ultra-cold polar molecules as qubits—the fundamental units of information that enable the power of quantum technology. The research, recently published in the prestigious journal Nature, signals a new era in quantum computing where the intricate internal structures of molecules can be harnessed to perform operations that could enhance computational speed and efficiency.
Senior co-author Kang-Kuen Ni, a leading figure in chemistry and physics, expressed the significance of this achievement, saying, “As a field, we have been trying to do this for 20 years, and we've finally been able to do it!” With quantum computing poised to revolutionize fields such as healthcare, scientific research, and financial systems, these developments are timely and essential.
The conventional realm of quantum computing has relied on trapped ions or superconducting circuits to implement quantum gates. However, the Harvard team took a more complicated route to devise a molecule-based iSWAP gate, a crucial quantum circuit that engenders entanglement, the powerful phenomenon at the heart of quantum computation.
In their groundbreaking experiment, the researchers expertly trapped sodium-cesium (NaCs) molecules using optical tweezers in an ultra-cold environment, leveraging the electric dipole-dipole interactions between the molecules to execute a quantum operation. By meticulously controlling the rotational relationships between the trapped molecules, they successfully created a two-qubit Bell state with an impressive accuracy of 94 percent.
What sets quantum gates apart from their classical counterparts is their ability to operate on qubits, which can exist in multiple states at once—unlike traditional binary bits (0s and 1s). This unique characteristic of quantum gates allows for complex computations that transcend the capabilities of classical computers, laying the foundation for new technologies and applications yet to be imagined.
The iSWAP gate implemented in this experiment not only swapped the states of two qubits but also performed a phase shift—an essential step in generating entangled states that remain correlated over distance, adding a layer of depth to quantum computing.
Co-author Annie Park highlighted the importance of their findings, calling it “a milestone in trapped molecule technology and the last building block necessary to build a molecular quantum computer.” The unique attributes of these molecules, including their rich internal structures, offer countless opportunities to refine and expand the potential applications of quantum technologies.
Although the idea of employing molecular systems for quantum computing has been around since the 1990s, early experiments faced challenges due to the inherent instability of molecules. However, by confining these molecules in ultra-cold conditions, the researchers successfully managed to minimize their unpredictable movements, ensuring that the delicate quantum states required for reliable operations could be maintained.
The effort that led to this monumental achievement involved a collaborative team, including members of Ni’s lab and physicists from the University of Colorado's Center for Theory of Quantum Matter. Their meticulous evaluations of the two-qubit Bell state and the errors associated with any residual motion has provided valuable insights for enhancing the stability and accuracy of molecular quantum operations in future experiments.
Excitingly, Kang-Kuen Ni remarked, “There's a lot of room for innovations and new ideas about how to leverage the advantages of the molecular platform. I’m eager to see what comes next!”
This breakthrough not only marks a significant leap forward in quantum computing but also beckons a future filled with potential applications that could redefine our technological landscape. Stay tuned as we continue to follow this exhilarating journey into the realm of molecular quantum computing!